
Chinese hamster ovary (CHO) cells attached on macrocarriers extracted from the iCELLis bioreactor fixed bed
Human and veterinary vaccines are divided into five main categories: conjugate, toxoid, subunit, inactivated (killed), and live (attenuated) vaccines (1). The vast majority of currently licensed human and veterinary vaccines are inactivated or live (2, 3). They are produced mostly using adherent cells: primary cells such as chicken embryo fibroblasts (CEF), human diploid cells such as MRC-5, or continuous cell lines such as Vero and MDCK (4). The pioneering legacy inherited by vaccine manufacturing development has led to strategies for cultivation of those anchorage-dependent cells. Those strategies rely on two approaches: either two-dimensional (2-D) systems (e.g., T flasks, roller bottles, Cell Factory, CellSTACK, and other stacked systems) or microcarrier cultures in stirred bioreactors.
Two-dimensional supports are quick and easy to implement with limited expertise. But scaling them up (by multiplying the number of systems) raises the issues of large footprint, capital investment, and operator requirements as well as high contamination risks due to a large number of open operations. On the other hand, microcarrier processes offer a more elegant solution at large scale. Such systems have limited footprint (high surface-to-volume ratio achieved by increasing microcarrier concentrations), lower labor requirements, and reduced contamination risk. But the expertise and time required (up to three years for >1,000-L scale) to develop a microcarrier process can be a time or financial barrier for some companies.
The fast-growing human vaccine market and the segmented veterinary vaccine market (together worth $27 billion) keep increasing demand for higher capacity from current manufacturers while also attracting a variety of new biotechnology and pharmaceutical companies (5, 6). All those groups usually face aggressive timelines and scale-up needs with financial constraints that standard 2-D systems and microcarrier cultures do not address. Fixed-Bed Bioreactor
ATMI LifeSciences wanted to solve those challenges and offer an efficient and cost-effective way to scale up a process of adherent cell culture while keeping footprint, labor, and expertise demands low. So the company developed a scalable fixed-bed disposable bioreactor: the Integrity iCELLis system.
This technology offers up to 500 m2 of individual, nonwoven, hydrophilized polyethylene terephthalate (PET) macrocarriers (11.2 cm2 each) compacted in a 25-L doughnut-shaped fixed bed. The matrix is incorporated in a 59- to 71-L working volume bioreactor that provides (through an internal centrifuge pump) a homogeneous distribution of media and cells throughout the fixed bed. Efficient gas transfer (high kLa values and CO2 stripping) is enabled by an internal, proprietary “falling film” system. The surface area of the 500 m2 fixed bed, is equivalent to 2,941 roller bottles (1,700 cm2), 794 10-tray Cell Factory systems (Thermo Scientific), or a 380-L stirred bioreactor with 3g/L of Cytodex 1 microcarriers (GE Healthcare).
With the iCELLis system, ATMI intends to tackle the issues that have historically prevented fixed-bed technology adoption: lack of scalability, insufficient oxygenation, no access to cells during culture, and the presence of cell or metabolite gradients within a fixed bed (7). Although the internal centrifugal pump and the falling filmensure an adequate distribution of media and gas transfer, the key for an efficient control of cell and metabolite gradients are the chromatography-like scale-up strategy. When scaling up processes from the small-scale iCELLis nano system (0.53–4 m2) to large scales (66–500 m2), media linear speed and fixed-bed height are maintained while bed diameter is increased (5–71 cm). A biomass probe allows cell density monitoring at large-scale.
A fixed-bed technology offers the ability to reduce initial seeding densities from two to 10 times those of 2-D supports. Biomass multiplication occurs in the fixed bed so that the seed train is significantly reduced. In the meantime, because of high oxygenation capacity, cell growth in fixed-bed bioreactors can lead to very high cell concentrations. A typical range of densities observed in the iCELLis bioreactor for Vero, HEK293, or MDBK cells is 30 to 100 × 106 cells/mL (8). Simplifying Development Steps
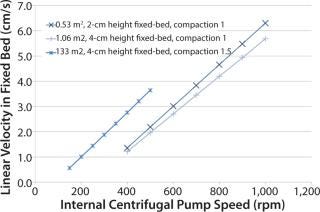
Figure 1: ATMI determined linear velocity inside a fixed bed at all the scales to facilitate determination of centrifugal pump speeds to apply when scaling up. Three scales were used in case study 1.
A fixed-bed system can simplify process development steps and reduce the cost of production when compared with 2-D supports or microcarrier-based processes. For instance, although a large-scale manufacturing process using roller bottles requires many operators and long operation times, a fixed-bed bioreactor can be operated by one or two people. Use of tube welders and sealers or sterile connectors also reduces contamination risk when compared with making multiple aseptic operations under a hood for roller bottles. Expanding roller-bottle capacity requires increasing the number of bottles. That leads to additional warm rooms or cleanrooms with multiple incubators and biosafety cabinets, which can represent a significant capital investment. But a fixed-bed bioreactor requires <2-m2 footprint for a controller with one tank for feed-in and one for feed-out that could fit in a limited space (<6 m2) (Photo 1). It would also require minimal investment for hardware.
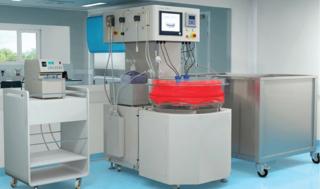
Photo 1: An iCELLis 500 bioreactor in operation mode. The temperature unit control, the controller and the perfusion in and out tanks require a limited footprint.
Microcarriers in disposable, stirred bioreactors also represent a smaller-footprint option with limited contamination risk. But the overall process requires more operations (microcarrier preparation and sterilization) than with a fixed bed, and perfusion is achieved by time-consuming media exchange using gravity to settle carriers in a bioreactor or with an external perfusion device that must be sterilized independently.
Developing a large-scale microcarrier process requires a lot of expertise and can take from two to three years — mainly because adequate conditions (shear stress) must be identified. Protocols for attachment, growth, and passaging steps (9, 10) represent major barriers to some companies. Choosing a disposable fixed-bed platform for scaling up from roller bottles or multitray processes reduces process development challenges and time, thereby ultimately reducing production costs. Case Studies
To illustrate the rationale mentioned above and demonstrate the reality of this model, three case studies were conducted as described below.
Case Study 1 involves transfer and scale-up of an adherent-cell–based process for viral vector production (8). The first step consisted of transferring an existing process from a multitray system to the iCELLis nano scale (0.53 m2). For this culture, multitray conditions were mimicked in the bioreactor to confirm that similar or higher cell-growth rates could be achieved. ATMI scientists maintained temperature, feeding strategy, media volume per surface ratio, and seeding cell density (cells per cm2) and based pH and dissolved oxygen (DO) set points on process background and literature.
The analysts dedicated a series of cultures to optimize cell growth and virus production conditions by testing various linear velocity, fixed-bed height and compaction, and feeding strategies until cell growth and virus productivity were satisfactory. That led to a process at 1.06-m2 (40 mm fixed-bed height) scale that was ready for scale-up.
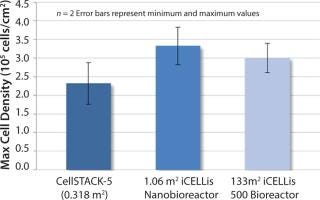
Figure 2: HEK293 cell density reached in CellSTACK-5 and iCELLis bioreactors (1.06 and 133 m).
Scale-up to an iCELLis 500 system offered the same fixed-bed height and compaction (133 m2). Cell culture parameters that maintained constant include pH, DO, temperature, linear speed set points, seeding, and infection cell densities (Figure 2).
Figure 2 shows the cell density achieved in both iCELLis bioreactor scales and the 2-D control (CellSTACK-5 trays). Cell densities were 29–43% higher in iCELLis than they were in 2-D supports, and equivalent cell densities were reached at both bioreactor scales.
Case Study 2 presents the linear scale-up of a human vaccine production process using Vero cells and a lytic virus (8). This development included multiple cultures at various scales:
Fifty cultures at 1 m2 and 2.7 m2 fixed bed
Fifty cultures at 13.2 m2 fixed bed
Five cultures at 132 m2 fixed bed
Two cultures at 660 m2 fixed bed.
ATMI achieved similar growth rates and specific productivity from smallest to largest scale (660 m2 prototype, not commercially available), in which productivity was equivalent to that of a 600-L stirred bioreactor with 6 g/L of Cytodex microcarriers.
Case Study 3 is a study performed by Vibalogics, a European contract manufacturing organization. The model used for this study was a paramyxovirus grown on Vero cells in serum-free media.
They achieved a cell density of up to 270,000 cells/cm2, but the most notable outcome of this study was the increased surface productivity (virus per cm2) observed in the iCELLis nano bioreactor (2.7 m2). That resulted in an 11.2× increase in TCID50 productivity and 5.5× increase in viral count productivity (11). Part of this surface productivity increase comes from a higher cell density at infection (35–80% more cells/cm2 in the iCELLis system) and better control culture conditions (temperature, pH, and DO) in the bioreactor than in T-flasks. Specific productivity was also higher than with microcarrier cultures, which leads to the hypothesis that a fixed-bed, 3-D environment can favor higher virus productivity. Proven Streamlined Process
The vaccine manufacturer landscape is evolving rapidly to address a rising global demand for higher volumes of existing vaccines and vaccines for new indications (6, 12, 13). Part of the equation to shorten times to market and cost effectiveness is to use more efficient manufacturing processes and shorten time requirements for process development. A disposable, fixed-bed technology such as the one described here offers a fast-to-market, more cost-effective alternative to traditional 2-D supports or microcarrier processes.
ATMI, Integrity and iCELLis are trademarks or registered trademarks of Advanced Technology Materials, Inc. in the United States, other countries, or both. All other names are trademarks of their respective companies. Individual results may vary.
About the Author
Author Details
Fabien Moncaubeig is senior application specialist and cell culture lab associate at ATMI LifeSciences, 10851 Louisiana Avenue South, Bloomington, MN 55438; 1-952-829-4460; [email protected].
REFERENCES
1 What Is a Vaccine? National Institutes of Allergy and Infectious Diseases; www.niaid. nih.gov/topics/vaccines/understanding/pages/ typesvaccines.aspx.
2 Complete List of Vaccines Licensed for Immunization and Distribution in the US. US Food and Drug Administration; www.fda.gov/ BiologicsBloodVaccines/Vaccines ApprovedProducts/UCM093833.
3 Veterinary Biological Products: Licensees and Permittees. US Department of Agriculture; www.aphis.usda.gov/animal_ health/vet_biologics/publications/ CurrentProdCodeBook.pdf.
4 Aunins JG. Viral Vaccine Production in Cell Culture. Encyclopedia of Industrial Biotechnology: Bioprocess, Bioseparation, and Cell Technology. Flickinger MC, Ed. Wiley: Hoboken, NJ, 2010: 1–35.
5 Wright B. Takeda’s Approach to Becoming a Global Player in the Vaccine Market. Life Sci. Leader August 2013: 24–29.
6 Kaddar M. Global Vaccine Market Features and Trends. Workshop on Business Modeling for Sustainable Influenza Vaccine Manufacturing. Washington, DC, 14–16 January 2013.
7 Pörtner R, Barradas O. Cultivation of Mammalian Cells in Fixed-Bed Reactors. Animal Cell Biotechnology. Methods Biotechnol. 24, 2007: 353–369.
8 Knowles S, et al. Linear Scalability of Virus Production in Integrity iCELLis Single- Use, Fixed-Bed Bioreactors from Bench Scale to Industrial Scale. ESACT 2013. Lille, France, 23–26 June 2013. www.atmi.com/ls-assets/pdfs/ bioreactors/icellis/iCELLis%20Poster_ Industry%20Scale_A4_Printer%20File.pdf.
9 Rappuoli R. Cell-Culture-Based Vaccine Production: Technological Options. The Bridge 36(3), 2006.
10 Venkat RV, Chalmers JJ. Characterization of Agitation Environments in 250 mL Spinner Vessel, 3 L, and 20-L Reactor Vessels Used for Animal Cell Microcarrier Culture. Cytotechnology 22(1–3) 1996; 95–102.
11 Lehmann S, et al. Evaluation of Fixed- Bed, Disposable Bioreactor Technology for Virus Production (Paramyxovirus); http://www. vibalogics.com/services/production-systems.
12 Carlson B. The Expanding Vaccine Market. Pharm. Process. 16 January 2012.
13 The Vaccine Industry in Figures; www. vaccineseurope.eu/about-vaccines-europe vaccines-europe-in-figures.