Highly Concentrated Protein Formulations: Finding Solutions for the Next Generation of Parenteral Biologics
May 1, 2014
Therapeutic protein formulation is no easy task. Biological drugs may be destined for prefilled syringes or glass vials, or they may be made into lyophilized powders that will be reconstituted in a clinical setting. No matter what their final state will be, recombinant proteins must remain potent and efficacious during storage. In recent years, pharmaceutical companies have turned increasingly to high-concentration protein formulations. Such drug formulations can offer patients the convenience of self-injection — instead of a trip to the hospital for an intravenous infusion treatment.
But these new formulations present a problem. Higher concentrations can exacerbate already existing tendencies for proteins to behave poorly. Such formulations are more likely to form aggregates that can reduce potency or even, perhaps, incite an immune-system responses. High-concentration formulations also tend to be more viscous, which can interfere with the plunging action of prefilled syringes. These principles apply to all proteins: monoclonal antibodies, vaccine components, enzymes, and so on.
“There’s no such thing as a well-behaved protein,” says Tom Laue, director of the University of New Hampshire’s Biomolecular Interaction Technologies Center.
Clinicians and patients are both pushing for subcutaneous delivery of biologic medications. Subcutaneous injection is easier to perform than intravenous injection as well as less expensive, and such drugs could be administered in a clinic or even at home, rather than in a hospital. A subcutaneous formulation must deliver the same dose of medicine in a 1-mL or 2-mL syringe that is delivered by a much higher volume of 250 mL or more in IV infusion — because intravenous formulations can be lower in concentration. Doses of 100 mg/mL or more are routine in the high-concentration field, but they can go much higher.
“Anecdotally, in some cases, I’ve heard clinicians asking for formulations containing 500 mg/mL of protein,” says Russell Burge, application scientist at Freeslate, which develops automated, high-throughput instrumentation. “Clinical data may support that the higher the concentration, the better the overall therapeutic effect (1,2,3). Naturally, they will push also for using something that has better patient adherence and is easier to administer, such as a prefilled syringe.”
Competition is increasing among biopharmaceutical companies to improve their drug formulations, especially for high-value indications. “It’s not enough anymore to have an effective drug,” says Jens Bukrinski, senior scientist at Novozymes A/S. “You also need to think about patient compliance and convenience.”
He cites an example of patients who may wish to travel — e.g., to visit family or on a planned vacation— even while they are being treated for chronic conditions such as cancer or multiple sclerosis. It may be impossible for them to visit a hospital every couple of weeks for a treatment. “If [a company] could provide a drug with three-month stability at 30 °C, that would be a competitive edge,” says Bukrinski. “Maybe even just a month: You could prepare your drug for a whole month and then bring it on vacation.”
Some of his company’s competitors believe this is a critical issue; Bukrinski remains unsure. “Maybe people would choose a less effective drug just to be able to lead a normal life,” he says. “At least it’s a risk that you need to consider.”
A Clinical Example
For an example of the competitive advantage that can come with a good formulation and delivery method, you need look no further than at drugs that target the proprotein convertase subtilisin/ kexin type 9 (PCSK9). This protein breaks down the low-density lipoprotein (LDL) receptors that otherwise remove excess cholesterol from the body. Pfizer, Amgen, Sanofi, and Regeneron all have clinical programs working with anti-PCSK9 antibodies. And Amgen recently announced that its drug reduced patients’ LDL by 55–66% in clinical trials. Alirocumab, in codevelopment with Sanofi and Regeneron, cut levels by around 47%. Those drugs are likely to be intended for patients who are unable to take statins or who have inherited high-cholesterol levels. Given the similar efficacy of the two antibodies, drug delivery is likely to be a key differentiator between them.
“The PCSK9 antibodies we’ve seen data for so far are not much differentiated in efficacy and safety,” Mark Purcell, an analyst with Barclays, wrote in a note to clients (4), “making dosing convenience a potential source of competitive advantage once multiple agents are on the market.” The companies are all developing injection devices that they hope will be easy for patients to use while minimizing injection volume.
Amgen is taking a dual track with a spring-loaded auto-injector and a disposable topical device. The latter will include an adhesive backing that allows patients to place it on their skin and then press a button to inject the drug. Sanofi is developing an autoinjection pen and a prefilled syringe, both of which it will be testing in clinical trials. Pfizer is also developing an autoinjector device.
Measuring Viscosity
by Lisa Newey-Keane (Malvern Instruments)
In biotherapeutics development, selecting the right candidate proteins from discovery involves a number of physicochemical testing processes intended to eliminate molecules that could have formulation, delivery, or manufacturing problems later on. Often only small amounts of material are available for these analytical tests, and parameters such as viscosity and physicochemical stability of formulations become critical quality attributes (CQAs) that determine whether a molecule is selected for further investment.
Because most biological molecules are unable to withstand the rigors of gastrointestinal delivery, most are administered through intravenous, intramuscular, or subcutaneous injection. Concentrations of the active molecules must be high — typically ≥100 mg/mL — to compensate for short plasma half-lives. One major challenge is to develop high-concentration formulations (for maximum effectiveness) that can be injected at low volume (for patient comfort). High concentration of biopharmaceuticals often creates high viscosity and potential stability/delivery problems.
Determining viscosity at a very early stage is therefore crucial. A new instrument (Viscosizer 200) for such measurements combines ultraviolet area imaging with microcapillary viscometry to deliver rapid, high-throughput, and nondestructive viscosity and molecular size measurements for biopharmaceutical formulations. It is an early development from the Bioscience Development Initiative established by Malvern Instruments. Through that program, Malvern partners with industry and academia to rapidly identify and assess analytical problems and bottlenecks within biopharmaceutical development and manufacture, then to identify, prototype, and deliver solutions (1).
For more details: www.malvern.com/bdi
And for more on viscosity measurement: www.malvern.com/biopharmdev
Reference
1 Lewis EN. How to Hit a Moving Target. BioProcess Int. 11(11) 2013: 42–46.
Other Applications: Even if the final drug product isn’t high in concentration, proteins can be at high concentrations during their manufacturing processes. For example, in ion-exchange column chromatography, the process-stream concentration may be kept high to reduce volume and speed up the purification process.
In fact, high protein concentrations aren’t just for clinical applications, according to Bukrinski. He notes that companies can generally achieve stable liquid formulations with protein concentrations up to 100 mg/mL. “That would be a fit for the purpose of most drugs I have seen in late-stage development and on the market,” he says. “In my view, the wish for very high protein concentrations comes from the development project. When you do your dose-escalation studies you need to go to very high doses, and then you are limited by volume to a much higher degree than you are in a commercial product.”
Managing Viscosity and Aggregation
First and foremost among the problems presented by highly concentrated formulations are viscosity and aggregation. “Those are the two very big issues that everybody talks about,” says Bukrinski. Protein characteristics that affect the potential for both viscosity and aggregate formation include hydrophobicity, charge patches and dipole interactions, and self-association.
Viscosity is a challenge for prefilled syringes, especially. “That has been a really big problem,” Bukrinski points out, “because some protein formulations are intended for disabled patients, and some of them would be unable to inject very viscous solutions.”
Burge agrees. “You just can’t go beyond a certain viscosity. Because they’re smaller than typical syringes, prefilled syringes need to have higher protein concentrations to meet dosage requirements, so their formulations have higher viscosities.” Viscosity also causes problems during manufacture and purification, for example by hindering filtration steps. “There, viscosity is a huge problem,” says Bukrinski.
Aggregation is always a key problem with protein formulations, and with high-concentration formulations in particular. It happens when two or more protein molecules bunch together, and the more concentrated the formulation, the greater the risk that it can occur. “As we push the concentration higher to reduce injection volume and produce better formulations,” says Elizabeth Topp, professor of industrial and physical pharmacy at Purdue University, “aggregation becomes more likely.”
Aggregated protein particles can gum up the works during purification operations, cause denaturation and thus affect clinical potency, or even prompt an immune response in recipient patients. “You want to make sure your drug does not associate in the formulation, or if it does, it does so in a reversible manner and dissolves relatively quickly,” says Laue.
A potential immune response could come in the form of neutralizing antibodies that make a drug ineffective. “Or maybe worse,” says Bukrinksi, it could cause an immune response. “You do see many high-concentration protein formulations that raise antidrug antibodies. We don’t know why, but it happens. One guess is that it’s because you have aggregation.”
Researchers are unsure whether the size of an aggregated particle makes a difference in the severity of its effects. Particles about 1 µm or larger have been well studied, and so far, no clear connection has emerged. “It’s not to say that [these larger aggregates] are safe,” Bukrinski explains, “but no connection is emerging like a jack-in-the-box, so there’s a push to [look at] smaller particles and see whether the problem lies hidden there.”
“We don’t have a good understanding of the properties of aggregates that can lead to an immune response,” Topp points out, “so it’s difficult to pin down what to measure.” In fact, disagreement remains over the significance of aggregates, at least when it comes to immunogenicity. Biotechnology industry representatives have argued that aggregates were a problem in products such as antibodies and blood isolates, the earliest of which were less pure than today’s biologics. Those early problems, in addition to more current data, have regulators and researchers such as Topp concerned that immune responses are still an issue.
One example is pure red cell aplasia (PRCA) apparently caused by aggregates in Johnson & Johnson’s Eprex erythropoietin product, which is used to treat anemia associated with chronic kidney disease (5). Before 1998, three reports suggested that the product could cause an immune response against the therapeutic protein that could cross-react with endogenous erythropoietin. In 1998, Johnson & Johnson modified the formulation for products marketed outside the United States, replacing human serum albumin as a stabilizer with polysorbate 80 and glycine. By 2002, the number of reported PRCA cases had jumped to 112, so the company withdrew the product from the market.
An analysis suggested that polysorbate 80 leached molecules from uncoated rubber stoppers in Eprex syringes. Those leached molecules may have acted as adjuvants, encouraging an immune response. More recently, two cases of PRCA arose as a result of subcutaneous administration of the biosimilar epoetin HX575 to patients with chronic renal failure during a premarketing trial. That problem may have occurred as a result of tungsten contamination during syringe manufacture. The metal contaminant may have caused denaturation and aggregation, which in turn could have caused an immune response (6).
Those incidents have “created a little bit of tension over the protein stability issue,” explains Topp. Industry representatives and regulatory officials remain at odds. “Both sides are right because we really don’t know [whether] aggregates are a significant problem in products.”
Measuring Aggregates: Such concerns have prompted the US Food and Drug Administration (FDA) and many biopharmaceutical companies to emphasize measuring aggregates in protein drug products, particularly focusing on the subvisible size range. Many analytical techniques are available for doing so, but they don’t produce consistent results across platforms. Methods include dynamic light scattering (DLS), resonant mass measurement, Coulter counting, microflow imaging (MFI, Figure 1), field-flow fractionation (FFF), atomic force microscopy, analytical ultracentrifugation, and nanoparticle tracking analysis.
Molecular Mechanisms
A protein’s solubility and viscosity both depend on proximity energies at particle interfaces. Higher concentrations reduce the interstitial spaces, increasing the effects of proximity energies. Attractive and repulsive forces affect the tendency for proteins to stick together. Van der Waals interactions are repulsive and operate at very close range. Hydrogen bonds and various dipole interactions are attractive forces that must be minimized to reduce aggregation and viscosity.
Two forces can be either attractive or repulsive, depending on circumstances. Charge–charge interactions are repulsive for same electrical charges or attractive for opposite charges. Desolvation energy involves expulsion of solvent molecules (typically water) from the space between two particles as they push closer together. On hydrophobic surfaces in an aqueous solution, desolvation can occur very rapidly, bringing surfaces close together and heightening viscosity. But in the presence of carboxyl groups, water molecules form hydrogen bonds with protein surfaces and stay put. The water molecules then cannot displace one another, which creates a buffer zone between proteins and discourages aggregation, keeping viscosity low.
“If you flank hydrophobic surfaces with negatively charged amino acids — such as an aspartate — then you’re going to trap the water in those surfaces,” Laue of the University of New Hampshire explains. Engineering a protein to include such residues may be a way to prevent aggregation “when you have a hydrophobic surface of a protein.”
Excipients such as detergents can reduce protein– protein interactions by binding hydrophobic areas. Increasing salt concentration of a solution weakens all electostatic interactions. So adding salt may increase aggregation by weakening charge–charge repulsion or decease it by weakening dipole attractions. Dipolar excipients (e.g., arginine) raise the dielectric constant and weaken all electrostatic interactions.
When it comes to limiting aggregation through protein design, Laue says, “charge is your only friend. If the protein has a low charge, you might as well just assume you’ll have trouble developing it.”
A protein’s charge is easily measured, but many companies simply calculate it based on isoelectric pH or X-ray structures. But those don’t always match reality. Laue cautions that they rely on some assumptions that aren’t always true. When his group compared calculated charges for IgGs with measured values, they found dramatically different results. “You think you know the charge by calculation,” Laue says, “and the fact of the matter is that it’s a phantom. You’ve got to measure the charge. At present, you cannot calculate the charge of a protein.”
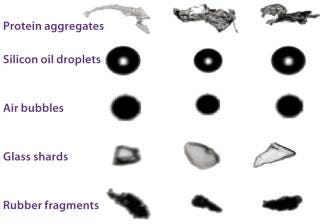
Figure 1:
“The methods can give conflicting results,” says Topp, “and no one really knows which is right. That makes it difficult to calibrate across instruments and makes the picture even more cloudy.” The problem is complicated by a lack of reference standards for protein aggregates in the relevant size range: roughly between 2 nm and 1 µm in size.
What’s more, researchers aren’t certain which attributes of protein aggregates determine undesirable effects in patients such as immune response. Is the size of an aggregate that’s the problem — or its shape — or some aspect of its surface chemistry? “We often measure size because we can,” Topp explains, “but what we’re really interested in is how the immune system responds.”
Larger particles can be detected using light obscuration, but this method is limited in range to those at least 1 µm in size. “It has been known for a long time that light-obscuration doesn’t catch all particles,” says Topp. At the other end of the scale, high-performance liquid chromatography (HPLC) is adept at measuring small particles up to 5 nm — from monomers and dimers up to about multimers of 10 proteins or so.
Championed by ProteinSimple, microflow imaging uses digital microscopy and microfluidics to collect images of particles in solution. The ProteinSimple system creates a database of particle count, size, transparency, and morphology. Particles can be accurately sized at 1 µm and larger, with morphology quantitative at 5 µm and larger. But there remains a gap between 100 nm and 1–2 µm for which few reliable technologies are available.
Some new techniques are making inroads into that range. Nanosight nanoparticle tracking analysis (NTA, Malvern Instruments) uses laser light-scattering to analyze particles in the 10- to 200-nm range. From a scattering pattern, the system’s software infers Brownian motion of particles, which in turn is used to calculate each particle’s hydrodynamic radius. The instrument measures each particle individually.
Another approach in this size range is resonant mass measurement, as represented by the Archimedes instrument, also from Malvern. According to the company, it detects and counts particles in the size range of 50 nm to 5 µm. As a sample flows through a microfluidic chamber, particles come into contact with a tiny cantilever that resonates with a specific frequency. The subtle shift in frequency caused by each particle allows calculation of that particle’s buoyant mass, dry mass, and size. Those traits allow the instrument to distinguish between protein aggregates and leachable contaminants such as silicone oil.
DLS can detect very small particles, but it can’t count them, and its results might be skewed because large particles can interfere with the light scattering of smaller particles. A mixture of particle sizes can muddy the results. On the other hand, techniques such as size-exclusion chromatography (SEC) and analytical ultracentrifugation can disrupt aggregates during the analysis. “What you measure may not be what you initially had in the sample,” says Topp.
Resonant mass measurement is designed to get around those limitations. It has no column to create shear stress that can disrupt aggregates, and it can measure particles one at a time, which eliminates interference. At high concentrations, unfortunately, interference can still occur when large and small aggregates crowd one another. And that must be solved by dilution, which can dissipate aggregates and thus lead to false-negative results. “There are limitations with all [the techniques],” Topp points out, “even the fancy new ones.”
Particle Sizing Systems is developing another method. Known as multiangle DLS, this technology grew out of the field of analyzing paint emulsions. According to the company, its system performs well in the 100-nm to 1-µm range, and it requires no dilution. Conducting DLS at a forward scattering angle increases measurement sensitivity. “It’s a completely new technology,” Bukrinksi says, “and [the company’s] claim is that it is extremely robust, which is not something we normally have. If it is half of what [the company] claims, I think it has a bright future.”
Robustness — that is, repeatability with reliable accuracy — would be a boon to laboratories that struggle with irreproducibility of aggregate measurements. It’s challenging because aggregates are affected by many factors, including solution pH and the size and number of dilutions.
Many techniques require at least one dilution, but that can disrupt aggregates, alter colloidal stability, and of course change viscosity and other key parameters. The sample that is ultimately assayed may not be fully representative of the original formulation it came from. As Burge explains, this problem has prompted analytical chemists at some pharmaceutical companies to call for eliminating or at least minimizing dilutions. “If you’re looking at aggregates and self-association events, once you dilute, you can lose a significant amount of information about those events.”
Researchers generally must define a particle concentration range before taking measurements, then calculate dilutions to reach an approximate concentration. Finally, depending on whether they believe the aggregates are reversible in their particular case, analysts must choose how long to wait before taking a measurement. “Oftentimes the analytical methods will decide how you do [the analysis],” warns Bukrinski, “not the science. And some of the methods are extremely low throughput. They can be used for characterization, but not [quality] control purposes.”
The desire for greater throughput has led to demand for automated systems, which also might reduce costs. Proteins are expensive to manufacture, and high-concentration formulations require more raw materials. “There’s a lot riding on the information [that formulators] are getting,” Burge explains. “Automation can reduce the amount of material that is used during formulation development.”
Automation’s small material requirements allow for testing of more formulations from a given manufactured batch. “It used to be that formulators would pick four formulations or so to move forward with,” he says. “But now with the high-concentration formulations, more testing may be necessary to ensure that the formulation in the final product is safe and effective, and more testing could also be necessary.”
Another key advantage to automation would be to reduce variability, which could be applicable to both formulation creation and analysis. “By removing variability among analytical chemists, we could improve the comparability of studies,” Burge says. “That’s an important advantage of automation.”
Containers: A Critical Decision
With all protein formulations, the choice of container is important. But it’s even more critical with high protein concentrations. Stoppers and needles might contain leachable components such as metals and synthetic polymers and additives that could seed particle formation or cause product degradation. Proteins can be the instruments of their own demise because they sometimes act like detergents.
Reconstituting Freeze-Dried Products by Cheryl Scott, BPI senior technical editor
At PepTalk 2014 in Palm Springs this past January, Wenjin Cao (Amgen, Inc.) presented “Mitigating the Challenge of Slow Reconstitutiton of Lyophilized High-Concentration Protein Formulations” based on teamwork with coauthors from Amgen, Gilead, Hospira, and Regeneron Pharmaceuticals. Cao explained that lyophilized highly concentrated protein formulations can take a long time to reconstitute, which can limit their practical use and commercial marketability.
Cao discussed a systematic approach to facilitating reconstitution of a 57-kD, 100-mg/mL Fc-fusion protein with stability issues that necessitated freeze drying. Many strategies can provide nominal improvement in reconstitution times: e.g., adding wetting agents to diluents, incorporating high-annealing steps in the lyophilization cycle, and reconstitution in a vacuum. Cao reports that reducing the diluent volume has significantly decreased reconstitution time by four to seven times for some highly concentrated protein formulations.
The team’s efforts to improve reconstitution time for their product included correlating lyophilized cake porosity with reconstitution time (and using diluents to shorten it); characterizing the lyophilization cycle (inducing mannitol crystallization to shorten reconstitution time); and exploring multiple reconstitution strategies (with half the fill volume and under vacuum). They found that reconstitution times correlate to cake crystallinity: Partly crystalline cakes reconstitute faster than amorphous ones. And highly concentrated formulations usually make for amorphous freeze-dried cakes — for which dissolution typically follows a slow erosion of the cake structure.
Ultimately, the team discovered that highly concentrated protein formulations need a lot of sucrose for sufficient stabilization. That translates to long reconstitution times, which made the formulations sensitive to reconstitution procedures, posing some difficulty in clinical settings. Some diluents (particularly high-concentration surfactants) reduced reconstitution time, but not significantly. Modifying the annealing step of the lyophilization process didn’t change crystallinity or reconstitution time much. But half-volume reconstitution did.
“If you increase the concentration,” says Murali Bilikallahalli, MedImmune’s associate director of formulation sciences for proteins, vaccines, and oligonucleotides, “there is more scavenging power. You tend to see more (leaching) at higher concentrations.”
“It’s disappointing to spend all this time and money to develop a useful drug,” Burge adds, “and when you put it into the final container, it doesn’t hold up its stability.”
Types of Container: High-concentration protein formulations can present difficulties when used with parenteral devices. When such a formulation encounters the surface of a plunger, a barrel lubricant, or contaminants left over from device manufacturing (e.g., tungsten particles), aggregation could result (7). Metals such as tungsten can leak from even treated rubber stoppers. Glass prefilled syringes can introduce silicone oil droplets, tungsten, and container components that negatively affect proteins.
“It can be as innocuous as a few droplets of oil that don’t harm the patient,” says Burge, “or these things can cause real problems for the proteins that could make them less safe or [reduce] their efficacy.” Leachables are a significant enough consideration that companies such as Becton Dickinson and Vetter Pharma (both of which design custom syringes, auto-injectors, and other delivery vehicles) include a leachables profile along with each device they develop.
Excipients can help, but only a few are applicable for reducing leachables in parenteral formulations. Metal chelators such as ethylenediaminetetraacetic (EDTA) and diethylenetriaminepentaacetic (DTPA) acids can assist formulators with these problems, as can antioxidant amino acids such as methionine or cysteine. But better devices would present an ideal solution. “There’s more emphasis built into the device side now,” Bilikallahalli says, “trying to come up with more compatible systems that can carry a number of biologic molecules such as vaccines, cell and nucleic-acid therapeutics, and proteins.” Some efforts to coat needle surfaces to prevent leaching are also in progress.
In recent years prefilled syringes have gained momentum within the biopharmaceutical industry. Burge describes an industry-wide move toward them.
Meanwhile, transdermal microneedles are also gaining interest. Some studies have suggested that transdermal administration can lead to better uptake into the lymphatic system than traditional subcutaneous injection provides (9, 10). More studies need to be done on a broad range of formulations, but Burge suggests that current data indicate that this mode of drug delivery could have great potential.
Materials: Containers can be made of glass or plastic. The latter is popular in earthquake-prone places such as Japan as well as with companies desiring lighter-weight inventory. High-concentration formulations increase the potential for material loss. “Japan lost hundreds of millions of dollars to the 2011 earthquake,” Burge reports. Plastic syringes don’t shatter easily, and they can have fewer [different] leachable components than glass. European and American companies still mainly use glass syringes, but Burge points out that many acknowledge the associated challenges (8).
Plastic has its own drawbacks. It can be permeable to oxygen, and some proteins are more likely to adhere to a plastic surface than a glass one, which can potentially lead to instability or degradation. Terumo Medical is a leading supplier of plastic syringes and has amassed great experience in working with proteins.
ADCs, Fusion Proteins, and Bispecific Antibodies
Antibody–drug conjugates (ADCs) face some unique formulation problems. The same degradation pathways are likely to be found for both naked and conjugated antibodies, but the linkers that bind toxins to antibodies can be troublesome. Those links are not always stable, and their failure can cause free protein to leak into solution. In some cases, this may necessitate a lyophilized formulation.
Linker technologies generally use either the side-chain amino group of a lysine or the sulfhydryl side chain of a cysteine. The latter is typically more stable in liquid formulations because the chemistry that targets cysteines is more specific than that targeting lysines. As a result, when lysine is used, off-target amino acids can be conjugated to the linker. In a liquid environment, those tend to form less-stable covalent bonds than does cysteine, according to Juan Davagnino, senior director of biopharmaceutical development at KBI Biopharma. Depending on the chemistry used by a given conjugation, the formulation group can determine whether a liquid or lyophilized formulation will be necessary.
ADCs also face solubility issues. The more toxins are bound to an antibody — the higher the drug–antibody ratio (DAR) — the more hydrophobic the final product tends to be, and that reduces solubility. The number of links per antibody inevitably varies, so each product is a mixture of molecules with different numbers of toxins attached. Those with more toxins tend to come out of solution first, another factor that often leads to lyophilization as the formulation of choice.
Because of those issues, ADCs are seldom used at higher concentrations. So ADCs are unlikely to be used subcutaneously, according to Davagnino. However, high amounts are still required for dose-escalation studies.
Other nontraditional biologics include fusion proteins and bispecific antibodies. Unlike ADCs, they mostly behave similarly to traditional proteins, Davagnino says. “I’m finding that the challenge is on the analytical (side). We have to develop new and sensitive methods to look at the different behaviors of these new constructions.”
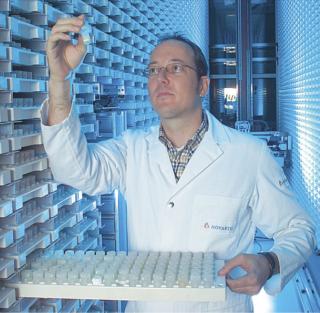
Formulation in BPI’s Online Archives
Other experts aren’t so sanguine. Kapil Gupta (senior fellow in integrated biologics profiling at Novartis Pharma), maintains that fusion proteins and bispecific antibodies have properties that are a mix of antibodies and therapeutic proteins, making them challenging to develop due to solubility problems that are seldom seen with antibodies. He has worked with both peptides and fusion proteins and calls them unpredictable. He also has found that such structures can be more prone to chemical modification and proteolytic degradation than are traditional proteins and antibodies. The excipients used to solve high-concentration challenges such as viscosity sometimes can worsen those problems. “You have to find a balance between the two,” Gupta warns. “It’s a unique challenge.”
Formulation in BPI’s Online Archives
Chen B, Zapata G, Chamow SM. Strategies for Rapid Development of Liquid and Lyophilized Antibody Formulations. BioProcess Int. 2(1) 2004: 48–52.
Scott C. Formulation Development: Making the Medicine. BioProcess Int. 4(3) 2006: S42–S56.
Garidel P, Schott H. Fourier-Transform Midinfrared Spectroscopy for Analysis and Screening of Liquid Protein Formulations. BioProcess Int. 4(5–6) 2006: 40–46; 48–55.
Apostol A, Kelner DN. Managing the Analytical Lifecycle for Biotechnology Products. BioProcess Int. 6(8) 2008: 12–19.
Simler R, et al. Maximizing Data Collection and Analysis During Preformulation of Biotherapeutic Proteins. BioProcess Int. 6(10) 2008: 38–45.
Maggio ET. Novel Excipients Prevent Aggregation in Manufacturing and Formulation of Protein and Peptide Therapeutics. BioProcess Int. 6(10) 2008: 58–65.
Zabrecky JR. Why Do So Many Biopharmaceuticals Fail? BioProcess Int. 6(11) 2008: 26–33.
Sharma DK, Oma P, King D. Applying Intelligent Flow Microscopy to Biotechnology. BioProcess Int. 7(6) 2009: 62–67.
Bursac R, Sever R, Hunek B. A Practical Method for Resolving the Nucleation Problem in Lyophilization. BioProcess Int. 7(9) 2009: 66–72.
Boehner R. A Formulation Strategy for Quickly Reaching Clinical Trials. BioProcess Int. 7(10) November 2009: 10–14.
Garidel P, Kebbel F. Protein Therapeutics and Aggregates Characterized By Photon Correlation Spectroscopy. BioProcess Int. 8(3) 2010: 38–46.
Woods JM, Nesta D. Formulation Effects on Opalescence of a High-Concentration MAb. BioProcess Int. 8(9) 2010: 48–59.
Scott C. Protein Conjugates. BioProcess Int. 8(10) 2010: 28–37.
Rios M. Combination Products for Biotherapeutics. BioProcess Int. 9(2) 2011: 27–35.
Wei Z, et al. The Role of Higher-Order Structure in Defining Biopharmaceutical Quality. BioProcess Int. 9(4) 2011: 58–66.
Mire-Sluis A, et al. Analysis and Immunogenic Potential of Aggregates and Particles. BioProcess Int. 9(10–11) 2011: 38–47; 38–43.
Reynolds G, Paskiet D. Glass Delamination and Breakage. BioProcess Int. 9(11) 2011: 52–57.
Scott C. Robots in the Laboratory. BioProcess Int. 11(10) 2013: 22–25.
Ridgway A, et al. Biosimilar Products. BioProcess Int. 11(10) 2013: 12–20.
Those issues call for extensive screening of different formulations, extended development timelines, and sometimes even novel excipients not used with most antibodies. At present, all these novel proteins are still in early development at several companies, so for now, higher concentrations will be needed largely for their dose-escalation studies.
The Role of Excipients
A number of excipients can be added to formulations to stabilize proteins by suppressing aggregation and surface adsorption. In liquid formulations, buffers with phosphate or histidine and a small amount of detergent can be useful. Excipients for lyophilized formulations include sucrose, trehalose, mannitol, and glycine, among others.
Other ingredients can be added to protein formulations to enhance stability. They fall into broad categories that include
protein stabilizers (to support a protein’s native conformation) including polyols, sugars, amino acids, and salts
hydrophilic polymers such as polyethylene glycol (PEG) and inert proteins that stabilize proteins and support protein assembly
surfactants that reduce protein aggregation and enhance protein refolding
amino acids that stabilize proteins through various mechanisms
microbial growth inhibitors such as benzyl alcohol.
Despite the challenges with protein aggregation in highly concentrated formulations, not much work has been done involving novel excipients, largely because they are difficult to get approved by the FDA. The agency doesn’t approve new excipients unless they are used in drug products, and then they are evaluated according to their intended use
“Big companies can do that,” Davagnino points out. “But in general, even the formulators in those companies tend to be quite conservative. The excipients we have tend to be good enough to produce good, stable formulations. I don’t see many newly approved formulations coming out with exotic excipients. It would have to be significantly better than what we have already.”
However, some researchers are pushing the field forward. Topp’s group is working on novel excipients designed to cover exposed hydrophobic residues on the surfaces of proteins. Their goal is to stabilize the native protein structure by preventing hydrophobic interactions that can lead to aggregation. These excipients — called artificial chaperones — mimic naturally occurring protein ligands, to act as a protective coating over aggregation-prone sites on the molecules.
Future Imperative
The challenges of highly concentrated protein formulations are not likely to be resolved any time soon. Most of these problems are solvable, however; formulation troubles rarely undermine a drug development program to failure. However, they can add time and expense to that program, and ultimately they could limit formulation applications for some drugs. But new excipients, technologies, assays, and containers should help manufacturers circumvent these potential roadblocks.
They’d better, Burge warns. “I don’t think the pressure from clinicians or from pharmaceutical companies is going to go away.” Highly concentrated formulations are the inevitable next generation of parenteral biologics.
Author Details
Based in Bellingham, WA, Jim Kling is a freelance science writer with a background in organic chemistry and over a decade of experience covering topics from biotechnology to astrophysics to archeology. His credits include WebMD, The Washington Post, Scientific American, Technology Review, and Science magazine. He also occasionally writes science fiction; [email protected].
REFERENCES
1.) Beck, A. 2010. Strategies and Challenges for the Next Generation of Therapeutic Antibodies. Nat. Rev. Immunol. 10:345-352.
2.) An, Z 2009.Therapeutic Monoclonal Antibodies: From Bench to Clinic, John Wiley & Son, Inc, Hoboken.
3.) Shire, SJ, Z Shahrokh, and J Liu. 2004. Challenges in the Development of High Protein Concentration Formulations. J. Pharm. Sci. 93:1390-1402.
4.) Armstrong, D, and MF Cortez. 2014.Amgen Drug Slashes Bad Cholesterol Within Weeks of Treatment Bloomberg News.
5.) Boven, K. 2005. The Increased Incidence of Pure Red Cell Aplasia with an Eprex Formulation in Uncoated Rubber Stopper Syringes. Kidney Int. 67:2346-2353.
6.) Seidl, A. 2012. Tungsten-Induced Denaturation and Aggregation of Epoetin Alfa During Primary Packaging As a Cause of Immunogenicity. Pharm Res. 29:1454-1467.
7.) Liu, W. 2010. Root Cause Analysis of Tungsten-Induced Protein Aggregation in Pre-filled Syringes. PDA J. Pharmaceut. Sci. Technol. 64:11-19.
8.) DeGrazio, F, and D Paskiet. 2012.The Glass Quandary Contract Pharma.
9.) Verbaan, FJ. 2007. Assembled Microneedle Arrays Enhance the Transport of Compounds Varying Over a Large Range of Molecular Weight Across Human Dermatomed Skin. J. Control. Release. 117:238-245.
10.) Wu, X, H Todo, and K Sgibayashi. 2007. Enhancement of Skin Permeation of High Molecular Compounds By a Combination of Microneedle Pretreatment and Iontophoresis. J. Control. Release. 118:189-195.
11.) Adler, M 2012.Challenges in the Development of Pre-Filled Syringes for Biologics from a Formulation Scientist’s Point of View Am. Pharmaceut. Rev.
12.) Alford, JR. 2004.PhD Thesis: Physical Stability of a Therapeutic Protein in High Protein Concentration Aqueous Formulations, University of Colorado, Boulder.
13.) Bhambhani, A, and JT Blue. 2010.Lyophilization Strategies for Development of a High-Concentration Monoclonal Antibody Formulation: Benefits and Pitfalls Am. Pharmaceut. Rev.
14.) Chi, EY 2012.Excipients and Their Effects on the Quality of Biologics AAPS J.
15.) Shire, SJ, Z Shahrokh, and J. Liu. 2004. Challenges in the Development of High Protein Concentration Formulations. J. Pharmaceut. Sci. 93:1390-1402.
You May Also Like