Getting Cell Therapy Right at the Source
February 8, 2024
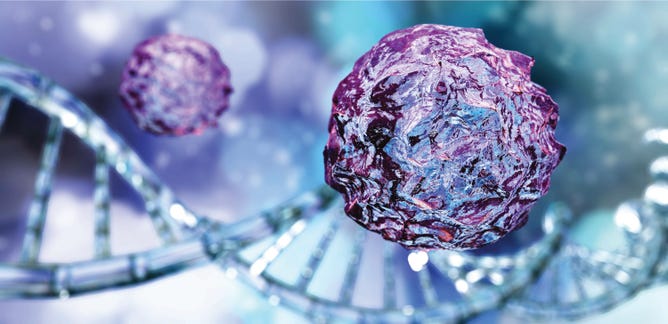
STOCK.ADOBE.COM
The materials used in cell and gene therapies (CGTs) come from human donors, introducing variability that complicates manufacturing. For consistency in product safety and efficacy, it is critical to address donor variability and optimize manufacturing at every step of CGT production. And that starts before cell collection.
Despite its rapid growth, the CGT sector has yet to coalesce robust, evidence-based standards in areas such as cell collection and donor screening. That is partly because many new technologies are in development in addition to already-approved materials such as chimeric antigen receptor (CAR)–T and pancreatic islet cells as well as enabling platforms such as clustered regularly interspaced short palindromic repeats (CRISPR) and viral vectors. To further complicate matters, donor-eligibility regulations differ by country, and there is bifurcation between requirements for autologous and allogeneic therapies (1).
The result is that developers — especially in early stages — may struggle to qualify their starting materials, leading them to develop narrow criteria that increase time and cost, leaving them unprepared to recruit donors. By understanding better how blood centers screen donors and collect and test cells, companies can optimize starting materials and accelerate their clinical use.
Automated apheresis enables efficient isolation of blood-derived starting material for advanced therapies, but it remains a time-consuming process with many variables that can affect a final leukopak product. The technology involves
• removing a specified volume of blood from a donor
• moving collected blood through a closed system with anticlotting agents
• separating components by centrifugation and/or filtration
• collecting target components in a leukopak
• returning the blood volume to the donor.
Developers must understand their starting-material needs when planning a donor screen, including biological and social screening criteria, preferred apheresis endpoint, target cell type and required volume, planned use of materials, and preferred shipping conditions. Perhaps the most important distinction is between autologous and allogeneic therapies, for which the apheresis processes differ greatly.
Getting the Most from Patient-Derived Blood
Apheresis is simpler in many ways for autologous products than for allogeneic products. Although baseline infectious-disease screenings are conducted in the same way in both processes, the resulting data are used differently. Because patients receive their own modified cells in autologous therapy, the presence of certain infectious pathogens does not preclude those cells from use. Instead, test results of starting materials and final products are compared postmanufacturing to ensure that the process has not been contaminated.
A patient’s apheresis experience will differ depending on developer specifications. In some cases, blood collection ends upon reaching a target count of the required cell type, which is typically highest for clinical-grade good manufacturing practice (GMP) materials. In other cases, blood centers might use different procedures, such as limiting the time that a patient spends being apheresed or using a predetermined number of filtrations for a certain blood volume. Testing for predonation leukocyte count and total blood volume helps blood centers determine how long collection will take.
Leukopaks can be filtered for specific cells on site. Depending on a developer’s need, collection can be broad enough to include all peripheral blood mononuclear cells (PBMCs) or narrow enough to include only T-cell subsets that express specific markers such as CD4 or CD8.
Apheresis sessions take several hours. Before the process, a blood center will either test a patient’s CD34+ cell count or perform a complete blood count with differential (CBCD) to assess readiness for the procedure and estimate the amount of blood needed to achieve a desired target. During apheresis, the blood center will perform a cell count from the leukopak itself to calculate the time needed to meet a target. An apheresis technician must monitor start and end times to keep from prolonging the procedure, which carries risk for both patients and collection processes. If collection does not meet target counts, the patient may need to return for an additional session.
Once a developer’s target is met, the apheresis site performs finished-product testing using, for instance, flow cytometry or CBCD. Results of a CBCD can help determine the quantity of cells and assess cell-type proportions. The goal is to ensure that the cells of interest have been collected with minimal contaminating cells, such as red blood cells and granulocytes. Additional quality control (QC) testing, such as for cell viability or sterility, is performed before shipping or processing to capture the percentage of viable cells in a leukopak.
Leukopaks can be shipped either fresh or frozen before manufacturing. Distance from collection to manufacturing site, travel logistics, and developer protocols dictate whether fresh shipments can be supported. Fresh leukopaks are shipped on the day of apheresis collection and typically are processed immediately upon receipt. Alternatively, leukopaks can be frozen ahead of manufacturing, and cryopreservation can occur either at the collection site or at a separate location.
When they are not manufactured fresh, leukopaks should be frozen within four to six hours of collection. A cryoprotectant such as dimethyl sulfoxide (DMSO) is added before leukopaks are cooled in a controlled-rate freezer to minimize cell death. The product is then shipped in a temperature-controlled container with a data logger that enables workers to monitor temperatures throughout the shipping process.
Allogeneic Adaptations
Allogeneic apheresis differs in part because the blood used during the process comes from healthy donors, which significantly reduces some variability observed in material from patients, who may be at advanced stages of disease. But for allogeneic therapies, developers are trying to understand the connection between baseline donor conditions and finished product quality. Thus, screening criteria are built on assumptions, especially regarding donor age and lifestyle.
Allogeneic apheresis products start with a CBCD. Unlike autologous products, almost all allogeneic leukopaks are cryopreserved for shipping to a manufacturing site, where they can be processed into material used to treat 100 or more patients with as many therapeutic doses. But although healthy donors can spend more time in the apheresis chair without health concerns, allogeneic therapies have their own complications.
When allogeneic therapies are intended for use in multiple jurisdictions, donor screening needs to comply with regulations for each geography. For example, Japanese regulators require that donors test negative for Epstein–Barr virus (EBV), but 90% of the world’s population has been exposed to that pathogen, complicating recruitment (2–4). A trial with an arm in Tokyo might need to apply such requirements across its entire clinical network. Different countries often have distinct rules about product pooling, a situation that can necessitate additional testing and donor categorization depending on the presence of protein markers such as human leukocyte antigens (HLAs).
Therapeutic developers often seek criteria that are rare among potential donors, and the pathway from a prospective donor to a collected leukopak requires careful management. That can include extensive screening and associated costs, necessitating that researchers balance the desire for specific donor characteristics against program-success criteria — particularly when considering the long-term needs for starting-material scale-up.
Another consideration is donor recallability. The field remains uncertain about some of the factors that determine the efficacy of certain donors’ cells. Developers may want to recruit “super donors” who can give PBMCs for multiple production runs. Donor characterization for cells of interest using flow cytometry can help identify super donors who can provide starting material that is especially well suited for therapeutic development. Once such donors have been identified, developers can ask them to return for additional collections. A well-characterized donor registry facilitates targeted recruitment, but large-scale donor screening could be necessary to identify super donors.
Standardization and Optimization
Several industry trends are changing the needs of advanced-therapy developers, increasing the urgency of developing collection standards to optimize product quality. Autologous therapy manufacturing is shifting toward decentralized operations that could change the way leukopaks are managed postcollection. And as more allogeneic approaches enter clinical testing, subtype varieties are increasing, making cell-selection processes even more important. Such subtypes include γδ T cells, which lack immune markers and must be filtered cleanly from both the more populous αβ T cells and natural killer (NK) cells, which all share a similar phenotype.
Therapeutic developers may decide to refine their screening criteria as clinical data come in. Blood centers often are essential partners in this process. They can support additional characterization and testing of donors and assess implementation of new criteria. Although well-versed in protocols, some blood centers also can identify nonoptimal processes and advise companies on how to refine their collection protocols. The right partners start with the right questions: Would increasing the donor age cutoff make it easier to find a suitable donor? Do existing data support restrictions such as cytomegalovirus-negative–status criteria? If time requirements are strict, should we expand the potential population by adjusting the body mass index (BMI) cutoff?
Savvy developers express a growing interest in adaptive protocols, and blood centers are well positioned to advise on characteristics based on their experience in apheresis. Such protocols might include establishing decision trees for troubleshooting and built-in contingencies. For example, developers could introduce alternative processes when clumping occurs in an apheresis product or additional methods to meet cell-count targets within optimal apheresis times.
Large-scale genomic and population health studies, especially the National Institutes of Health (NIH) All of Us Research Program, can provide broad insights and guide standardization efforts. Such programs better elucidate factors that influence health, and such data someday could be used to correlate inputs from a patient or donor with therapeutic outcomes.
That correlation will be key for the CGT field to mature fully. Apheresis provides bedrock starting material for advanced-therapy manufacturing. By increasing developers’ understanding of the factors that have the biggest impact on safety and efficacy, process optimization at the earliest stages of development will enable better outcomes for tomorrow’s patients.
References
1 Kuker B. International Differences in Quality and Regulatory Requirements for Cellular Starting Material. BioProcess Int. 22(7–8) 2023: 32–35; https://bioprocessintl.com/2023/july-august-2023/international-differences-in-quality-and-regulatory-requirements-for-cellular-starting-material.
2 MHLW Ministerial Announcement No. 210. Standards for Biological Ingredients. Ministry of Health, Labour and Welfare of Japan: Tokyo, Japan, 2014; https://www.std.pmda.go.jp/stdDB/Data_en/InfData/Infetc/06_Criteria.pdf.
3 PFSB/MHLW Notification No. 1314 Appendix 1. General Principles for the Handling and Use of Cells/Tissue-Based Products. Pharmaceutical Devices Agency: Tokyo, Japan, 2000; https://www.pmda.go.jp/files/000205395.pdf.
4 Womack J, Jimenez M. Common Questions About Infectious Mononucleosis. Am. Fam. Physician. 91(6) 2015: 372–376; https://pubmed.ncbi.nlm.nih.gov/25822555.
Corresponding author Becky Cap is senior vice president, advanced therapies, BioBridge Global, 6211 Interstate Highway 10 West, Building 1, San Antonio, Texas 78201; For inquiries, contact [email protected].
You May Also Like