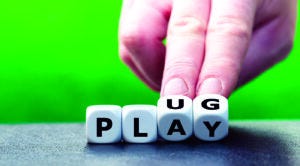
HTTPS://STOCK.ADOBE.COM
Discovered in the mid-1960s, adenoassociated viruses (AAVs) have become the leading vector for gene therapy in recent years. In October 2012, the first European market authorization for a human gene-therapy product was granted for UniQure’s Glybera (alipogene tiparvovec), which contains an AAV1 vector for treating patients who have lipoprotein lipase deficiency. (The product has been withdrawn from the market because of limited demand.) Both gene therapies currently approved in the United States — Luxturna (voretigene neparvovec) from Spark Therapeutics, approved in 2017, and Zolgensma (onasemnogene abeparvovec) from Novartis, approved in 2019 — are AAV-based gene therapies. Most ongoing gene-therapy pipelines remain focused on treating monogenic and rare human diseases. Some patients already have realized great benefits even as these programs advance our understanding and bring hope for those with complex diseases.
An AAV’s protein shell surrounds and protects its small (25-nm), single-stranded DNA genome of ~4.7 kilobases (kb). That genome contains just three genes: rep (for replication), cap (for the capsid) and aap (for particle assembly). Lacking viral DNA, recombinant AAV (rAAV) is essentially a protein-based nanoparticle engineered to pass through cell membranes, through which it carries and delivers a therapeutic DNA “cargo” into the cell’s nucleus (1). Because AAVs cannot replicate without outside help, they provide a safe vehicle to drive long-term transgene expression after a single infection. These vectors package only single-stranded genomes that require host-cell synthesis of the complementary strand for transduction. However, most people already have been exposed to wild-type AAVs and thus have preexisting adaptive immunity against these viruses. Sometimes that can undermine the drugs’ clinical efficacy and prevent readministration.
AAVs are considered to have ideal characteristics for gene-therapy development because of their replication defectiveness, low immunogenicity in humans, and inability to integrate into a host-cell genome. Their own simple genomes also make these viruses versatile and easy to engineer. Their primary limitation is the packaging size (~4.7 kb) of recombinant DNA that they can carry.
Market Trends and Needs
Recent successes in commercializing AAV gene therapies have increased interest in cell and gene therapy greatly over the past few years. Globally, more than 2,400 CGT clinical trials are ongoing currently (2). In addition, CGTs have shorter development timelines than those of traditional biologics and follow an accelerated regulatory approval pathway. The US Food and Drug Administration (FDA) has announced regenerative medicine advanced therapy (RMAT) designations for 66 products so far. Such accelerations shift the clinical trials paradigm and contribute to increasing demand for CGT manufacturing capacity. The gene, cell, and RNA therapy landscape continues to expand, with the gene-therapy pipeline alone having increased 16% since the first quarter of 2021. Currently, 3,579 gene, cell, and RNA therapies are in development from preclinical through preregistration stages (2).
As the CGT market shifts beyond ultrarare indications, viral-vector manufacturing requires rapid expansion to address a number of other diseases at commercial scales. Leading contract development and manufacturing organizations (CDMOs) such as AGC Biologics have invested heavily in this space (3). The increasing demand has required manufacturing technologies to evolve rapidly. Broadened application of viral-vectored gene therapies (e.g., to more common diseases) will require increased yields and decreased cost of goods (CoG).
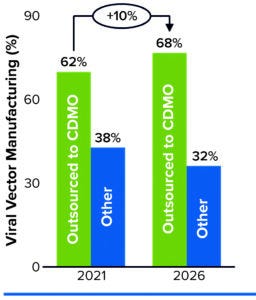
Figure 1: Predicting manufacturing service needs over five years.
In a February 2022 report, Kate Hammeke (vice president of market research at ISR Reports) cites survey results showing that, when it comes to outsourcing, the largest segment of respondents (44%) use CDMOs to augment their internal capabilities (4). About 25% have no CGT manufacturing capabilities themselves (but manufacture biologics or small molecules in house), and about 20% outsource all of their manufacturing. Outsourcing demand is highest for viral vectors, with 62% of respondents engaging a CDMO for related manufacturing capabilities. That need is expected to remain steady over the next five years, rising slightly to 68% by 2026 (Figure 1).
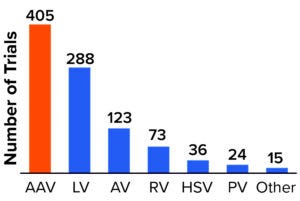
Figure 2: Viral vectors used for products in clinical development.
AAV = adenoassociated virus, LV = lentivirus, AV = adenovirus, RV = retrovirus, HSV = herpes simplex virus, PV = parvovirus.
AAVs are used in at least 46% of all gene-therapy trials — and 66% of those for which the viral vector is publicly known (3). As Figure 2 shows, 89% of gene therapies in development use viral vectors for delivery, with AAV and lentivirus (LV) vectors remaining the most frequently used.
Hammeke also points out that greater investments in technology and infrastructure are needed to support scalability. Responses cited in the report include “being able to take the product from bench to market for the client” and “being able to scale up rapidly in a cost-effective manner.”
Viral vector manufacturing is not standardized across the industry. Different biopharmaceutical companies use different production systems and downstream processes. In some cases, a single company might use diverse systems across its portfolio (3). My company’s research has found no standard, off-the-shelf production platform for clinical and commercial vector manufacturing. Establishing such a platform could minimize development efforts and complexities, thus helping to accelerate good manufacturing practice (GMP) production of materials for clinical trials.
Toward Scalable AAV Platforms
Some approaches have potential for creating platforms that could address CDMO market challenges related to CGT manufacturing, specifically for AAV-based gene therapies.
Scope of Manufacturing: A number of manufacturing systems are used to produce AAV vectors. Those include a plasmid-transfection system for human embryonic kidney (HEK293) cells, the baculovirus system for insect cells, and the helper-virus system using herpes simplex virus (HSV) or adenovirus (AV) for producer cell lines. Each system presents its own advantages and limitations in flexibility, scalability, and quality. Choosing a manufacturing system relies heavily on developers to evaluate and select among those trade-offs at an early stage based on both the proposed market landscape and the size of the potential patient population.
My company focuses on the most common vector system: transient transfection using HEK293 cells. We use two leading cell-culture technology platforms to manufacture AAVs for gene-therapy product pipelines: suspension and adherently growing cells. Both approaches use the human-derived HEK293 cell line and three transiently transfected plasmids: a rep/cap plasmid, a recombinant vector-genome plasmid, and a helper plasmid that expresses adenoviral genes. Before transfection, the manufacturing process is product independent, thus enabling multiple products to be made by changing the transfection step depending on the gene of interest (GoI).
Adherent Platforms: Most cell lines, especially those of mammalian origin, grow adherently. Because those are readily sourced, early gene-therapy pipelines made adherent-cell technology their primary choice. For example, my company cultures adherent HEK293 cells first in Corning HYPERFlask vessels, then transfers those cultures to seed a Pall iCELLis 500 bioreactor for production. There they progress through DNA transfection, addition of Benzonase reagent (MilliporeSigma), and cell lysis. Bulk harvest material is passed through a depth filter, then gets concentrated to a desired volume. After diafiltration, retentate is concentrated further and then harvested into a collection bag.
At that point, the process intermediate can be frozen as needed according to production schedules. Later, the thawed material continues to downstream processing, beginning with clarification and filtration. Ultracentrifugation or ion-exchange chromatography (IEC) technology can be used to separate empty and full capsids. Viral vectors are purified and captured into a final formulation. Drug substance can be frozen at this point as well. The last steps are sterile filtration and filling into the final drug-product containers.
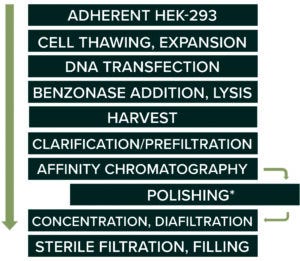
Figure 3: An adherent-cell–based platform process for AAV production.
* serotype dependent
Figure 3 demonstrates an adherent platform approach. A number of technologies are available: Thermo Fisher Scientific’s Cell Factory (CF) systems can provide up to 25,100 cm2 of attachment surface in 40-tray stacks; Corning HYPERFlask vessels provide a surface of 1,720 cm2 in a 175-cm2 culture flask. Providing a surface area of up to 500 m2, Pall’s iCELLis 500 single-use fixed-bed bioreactor system is used most often for large-scale AAV manufacturing with adherent cells.
Adherent culture technologies are relatively mature and thus widely used in both clinical and commercial AAV manufacturing. The platform has demonstrated many benefits, including consistent yield, reliable transfection efficiency, and so on. However, the cell-expansion process is labor-intensive, with multiple passages handled manually. This approach impedes progress toward lowering CoG and improving overall process yields. Because the process relies on a bioreactor’s culture surface, scalability has become a major, well-known limiting factor. Often, to increase process yield and meet the total dose requirements, multiple upstream batches are pooled to make one downstream batch. That complicates quality control and increases process cost.
Organizations can optimize the process by implementing a scale-out strategy for adherent-cell platforms to maximize yields. At our site in Longmont, CO, we have had success running six iCELLis 500 bioreactors in parallel and maintaining a seventh unit as a back-up.
Despite requiring a large facility footprint and significant capital investment, a scale-out approach for adherent-cell cultures can provide a reliable means to a “first-to-market” manufacturing strategy. For many AAV products currently in the clinical stage (especially those targeting rare diseases), that would be the greatest contributor to commercialization success. The scale-out strategy minimizes redevelopment requirements and can support rapid commercialization without complicating regulatory filing. Both FDA-approved AAV products mentioned above rely on adherent-cell technology in manufacturing.
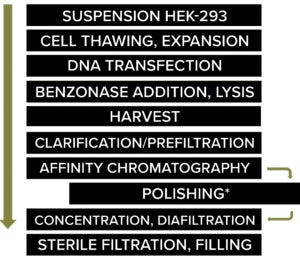
Figure 4: Suspension culture process.
* serotype dependent
Suspension Platforms (Figure 4): Many mammalian cell lines, including HEK293, can be adapted to grow in suspension. As the scale of production becomes larger for gene therapies, suspension culture technologies are emerging as an alternative. I recommend implementing a full range of bioreactor systems and sizes. For example, Ambr 10 and 250 systems from Sartorius provide options for product development, whereas commercial manufacturers can use 50-L, 200-L, 500-L, and/or 2,000-L single-use stirred-tank XDR bioreactors from Cytiva.
Although suspension-based platforms are relatively well-established in traditional biologics production, the technologies are still in an early stage of development for CGTs. Note that nearly 60% of the 2,400 ongoing regenerative medicine trials at the end of 2021 targeted prevalent rather than rare diseases (2). The first gene-therapy approval for such a treatment in the United States and/or Europe could be only a few years away. The scale-out approach of adherent-cell technologies cannot supply the required number of doses for late-phase trials and commercial demands for prevalent-disease treatments.
Scalability is a significant advantage of suspension technologies. Such cell-culture vessels can be scaled up easily from shaker flasks to laboratory-scale stirred-tank reactors and then to a production-scale bioreactor that might have a total volume of 2,000 L or more. Suspension cultures also use serum-free media, which not only increase safety (by eliminating a potential contaminating source of adventitious agents), but also simplify subsequent downstream purification and reduce analytical testing requirements.
One drawback of suspension cultures comes with the hydrodynamic shear effects of stirred tanks, which can reduce cell growth and productivity. Few suspension-based CGT processes have been approved, and 70% of clinical-pipeline products still use adherent-cell technology (5). Switching from adherent to suspension-adapted cells probably would require a detailed and time-consuming comparability study, thus extending the regulatory filing timeline for a product in development.
Suspension-adapted cell lines for production of viral vectors will be crucial for large-scale manufacturing. Decades of work have gone into improving cell-line robustness for this purpose. My company’s site in Milan, Italy, developed an in-house suspension cell line beginning with HEK293 cells from the American Type Culture Collection (ATCC). The cells grow in a chemically defined medium without fetal bovine serum (FBS) and have been tested for AAV8 productivity. Insect cells also can be used in suspension processes, as is the case for UniQure’s Glybera gene therapy.
Many different AAV serotypes and both engineered and customized AAV vectors are available to help developers further enhance precision and delivery efficiency to targeted tissues. Note that downstream process difficulties can be amplified in large-scale vector manufacturing. Although ultracentrifugation can yield high-purity, high-potency vectors, scalability of such processes is significantly limited. Despite remarkable advances in purification of AAV vectors, the need to process large-volume process streams presents a challenge. Chromatography using affinity binding and/or ion exchange can provide efficiency, flexibility, and scalability. But such methods do not yet achieve the same degree of separation for empty and full capsids. In our experience, some downstream steps need to be fine-tuned to account for chemical and biological differences among different AAV serotypes.
Overall, however, a suspension-based platform should facilitate process scalability, simplify manufacturing, improve process productivity, and increase process economics and cost-effectiveness. A standardized suspension platform for gene therapies would benefit both early stage product development and scale-up to commercial production.
Platform Selection
Choosing a technology platform is a critical early stage decision that developers need to consider carefully. As discussed above, both adherent and suspension technologies offer benefits and drawbacks. The questions listed below can help guide the evaluation and selection process:
• What is the patient population for the targeted disease indication? Is scalability a potential bottleneck for commercialization?
• What cell lines and AAV serotypes will be used?
• What stage has process development reached? Can the project budget accommodate process optimization or a potential change in platform?
• What is the manufacturing strategy for clinical and commercial phases?
• Does the regulatory filing strategy prioritize a “speed to market” or “best in class” approach? Is a parallel strategy possible, and should that be considered?
Technology innovation is advancing rapidly in the CGT market. The COVID-19 pandemic has accelerated implementation of new technologies. We are entering an exciting era for advanced therapies that will require companies to choose the right technologies for balancing speed, cost, and quality.
Platform Approach or Customized Solution?
Despite the complex and dedicated manufacturing processes for CGTs, we believe that standardization is on the horizon. Biomanufacturers face many concerns — including CoG, market speed, and quality testing — that can be minimized with a standardized approach. The COVID-19 pandemic caused supply-chain constraints and highlighted the limitations of using customized single-use consumables, media, and buffers.
At my company, we believe that CDMOs could play an essential role in driving standardization of viral-vector manufacturing. Service providers can share or reduce the competitive CGT market risk and bear some associated burdens of significant capital investment for establishing in-house manufacturing capabilities. CGT developers — particularly, small companies — can leverage CDMO manufacturing platforms and both technical and regulatory expertise for accelerated programs. Collaboration among industry and regulators could encourage and facilitate the standardization of CGT manufacturing. With the help of emerging regulatory guidance, our growing collective knowledge should advance standardization much more than any individual company can imagine.
Platform approaches have helped other biologic products advance (e.g., monoclonal antibodies) by increasing downstream process recoveries to 90%. We believe that CGT downstream processes are likely to become increasingly standardized over time as experience in the industry grows and platform approaches are developed for the most common viral vectors. However, no one solution fits all needs. Some unique and complicated products will continue to require customized processes because they cannot be tailored to a standard platform. Effective advanced therapies are the ultimate goal for both developers and manufacturers.
References
1 Naso MF, et al. Adeno-Associated Virus (AAV) As a Vector for Gene Therapy. BioDrugs 31(4) 2017: 317–334; https://doi.org/10.1007%2Fs40259-017-0234-5.
2 Regenerative Medicine: Disrupting the Status Quo. Alliance for Regenerative Medicine: Washington, DC, 2021; https://alliancerm.org/?smd_process_download=1&download_id=11068.
3 Capra E, et al. Viral-Vector Therapies at Scale: Today’s Challenges and Future Opportunities. McKinsey & Company: Seattle, WA, 29 March 2022; https://www.mckinsey.com/industries/life-sciences/our-insights/viral-vector-therapies-at-scale-todays-challenges-and-future-opportunities.
4 Hammeke K. What’s Happening in Cell and Gene Therapy Development? Life Science Leader 1 February 2022; https://www.lifescienceleader.com/doc/what-s-happening-in-cell-gene-therapy-development-0001.
5 Pettit S, et al. Upstream Manufacturing of Gene Therapy Viral Vectors. Cell Culture Dish 20 January 2021; https://cellculturedish.com/upstream-manufacturing-gene-therapy-viral-vectors-2.
Maggie Chen is senior director of manufacturing for cell and gene therapies at AGC Biologics, 4000 Nelson Road, Longmont, CO 80503; 1-720-417-6822; https://www.agcbio.com. All registered trademarks mentioned herein are property of their respective owners.