Protein A affinity chromatography offers efficient monoclonal antibody (MAb) purification and is used extensively in large-scale MAb production. As is the case with most chromatography media, protein A resins often have some degree of nonspecific binding, which causes host-cell proteins (HCPs) to coelute with a MAb. To reduce nonspecific binding interactions, an intermediate wash step can be performed before product elution. Doing so can improve product purity, extend column lifetime, and potentially eliminate a subsequent polishing step. For large- scale purification processes, it can be worthwhile to optimize the wash and elution conditions to maximize yield. Intermediate wash conditions are often dependent on specific feeds with specific resins. This study showcases two specific CHO feeds with Eshmuno A resin.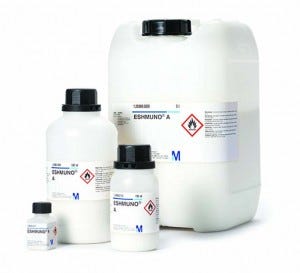
A wide range of intermediate wash conditions for protein A chromatography have been described elsewhere (1–5). Those include different pH and salt conditions, as well as the presence of various additives. Whereas some of the more effective intermediate washes contain additives, their disadvantages could include toxicity, corrosiveness, flammability, instability, and inefficient removal. We explored intermediate wash conditions for Eshmuno A affinity chromatography resin (EMD Millipore).
In addition to several pH and salt conditions, we studies additives such as arginine and guanidine hydrochloride (GuHCl). We chose those conditions to interrupt the undesirable interactions of HCPs to protein A resin or HCP to protein A– bound antibodies (6). More than 20 different combinations were screened using a 96-well plate format to determine the top candidates. The key parameters are HCP and yield from the elution samples.
We confirmed results from the well-plate study using laboratory-scale column tests. We then identified optimal intermediate wash conditions for Eshmuno A resin with two specific Chinese hamster ovary (CHO) feeds. Our study offers insight into process development strategies for the protein A chromatography step.
Materials and Methods Chemicals and Equipment: Buffer reagents came from EMD Chemicals and Thermo Fisher Scientific. The antibody was produced and purified in-house (within EMD Millipore facilities) with a titer >0.5 mg/mL. We measured Eshmuno A resin and dispensed it into the 96-well filter plates (Whatman clear polystyrene 0.45-μm hydrophilic PVDF filter). For column experiments, we used an ÄKTA Avant 25 system (GE Healthcare, Uppsala, Sweden) using resin that was packed into OmniFit columns (1 cm i.d. × 5 cm bed height).
Methods: Plate-screening studies were based on protocols established in literature (7, 8). Table 1 lists method details. The resin was equilibrated and then incubated at room temperature for 60 minutes with a MAb feed. We deposited the resin into 96-well plates in a 50% slurry and tested different intermediate wash conditions in parallel using this screening approach. We eluted the MAb from the resin and evacuated the liquid from the plate at each step using vacuum filtration (MilliBlot vacuum pump).
Table 1: Method details
We recovered supernatants in UV-transparent collection plates (Corning Costar 3635) for UV analysis. We screened each condition in triplicate for assay analysis. The chromatography method, steps, buffers, and column volumes used in the plate screening studies were also used in the subsequent column studies. We determined HCP content using Cynus Technologies F550 CHO HCP enzyme- linked immunosorbent assay (ELISA) kit 3G immunoenzymetric assay. Yield was based on UV absorbance at 280 nm.
Results and Discussion High-Throughput Screening: We selected intermediate wash conditions to sample a range of salt types, salt concentrations, pH levels, and additives (arginine, GuHCl, glycol molecules). The objective was to interrupt undesirable interactions of HCPs to protein A resin or HCP to protein A–bound antibody. We evaluated screening conditions based on their impact on product yield and HCP removal, using an equilibration buffer intermediate wash as a benchmark for this study. An intermediate wash consisting of equilibration buffer is not always optimized and cannot selectively disrupt the undesirable interactions of HCPs to protein A resin or HCP to protein A– bound antibody. Equilibration buffer is also unlikely to disrupt antibody-binding interactions to protein A, providing high yields.
Figure 1 shows the baseline set of data obtained by using equilibration buffer as intermediate wash buffer. The figure also shows a comparison with an intermediate wash consisting of 0.1 M citric acid, pH 5.5. This intermediate wash condition significantly reduced HCP levels in the final elution pool — below those obtained using an equilibration buffer wash.
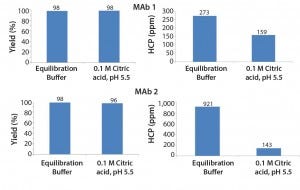
Figure 1: Effect of design decisions on potential savings and costs as a function of decision time
Figure 2 shows the results of different arginine concentrations in intermediate wash buffer. Arginine dramatically reduced the level of HCP in the elution pool while also contributing to high product yield. This result is consistent with literature findings (4). The level of HCP resulting from an equilibration buffer wash (50 mM Tris, 25 mM NaCl, 5 mM EDTA, pH 7.2) is indicated. Results shows that 0.1–1 M arginine in the intermediate wash improves HCP removal beyond that of an equilibration buffer wash.
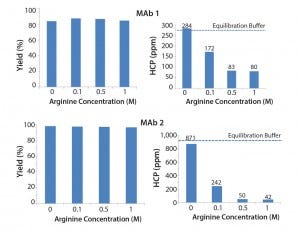
Figure 2: Effect of arginine on MAb yield and HCP removal
Figure 3 shows the effect of different GuHCl concentrations. We observed that increasing GuHCl concentration led to less HCP in the elution pool. However, increasing GuHCl concentration beyond 1 M does not offer further benefit and MAb yield begins to suffer at 6 M GuHCl.
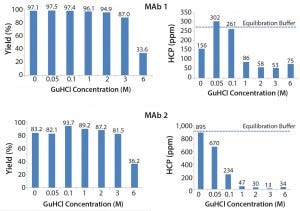
Figure 3: Effect of guanidine HCl on MAb yield and HCP removal
Figure 4 shows the effects of various NaCl concentrations and buffer pH levels. Results show that increasing NaCl concentration causes no significant change in HCP for both MAb feeds. We observed limited improvement with a low pH wash in these two MAbs and lower yield when pH reaches 4.
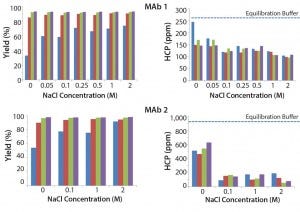
Figure 4: Effect of NaCl concentration and buffer pH on MAb yield and HCP removal; (blue) pH 4, (red) pH 4.5, (green) pH 5, (purple) pH 5.5
Based on results of the 96-well-filter plate screening, we selected four of the most promising conditions for further testing in column studies. Those conditions were chosen based on yield and HCP results. Figure 5 shows the four conditions. According to plate-screening data, the intermediate wash consisting of 50 mM Tris, 0.5 M arginine, pH 8.5 and 0.05 M Tris, 2 M GuHCl, pH 8.5 led to the lowest HCP levels in the elution pool for both feeds.
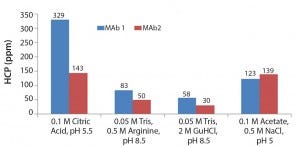
Figure 5: Four of the most promising intermediate wash conditions identified from the 96-well filter plate screening
Figure 6 shows the results of using the four selected intermediate wash conditions on laboratory-scale column testing. We observed the same trend as with plate studies, in which 0.05 M Tris, 0.5 M arginine, pH 8.5 and 0.05 M Tris, 2 M GuHCl, pH 8.5 led to the lowest HCP levels in the elution pool.
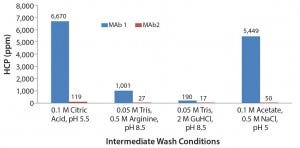
Figure 6: Column results of the four conditions identified from the 96-well plate screening
To account for the fact that the absolute numbers for the HCP levels in the column studies were different from those in the plate studies, we calculated those values as log-reduction values (LRVs) (Figure 7). Those results make it possible to compare the HCP removal despite the fact that the initial HCP levels were not identical.
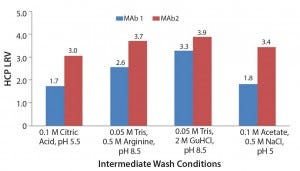
Figure 7: Log reduction values (LRV) for host-cell protein removal using Eshmuno A
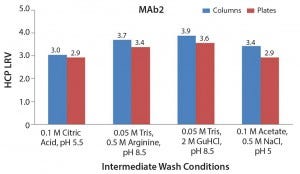
Figure 8: Comparison of host-cell protein removal values obtained by column and plate experiments
Comparing High-Throughput with Column Experiments When we compared LRVs for the column and plate experiments for the four selected intermediate wash conditions, we found consistent results. This further verifies that the 96-well filter plate approach is amenable to those types of screening activities.
By analyzing HCP levels and product yield resulting from a range of intermediate wash conditions, we identified several conditions that improve product purity. Significant HCP removal is observed by using citric acid at pH 5.5. In general, we observed a trade-off between purity and yield when using pH and salt intermediate wash conditions. Although low-pH intermediate wash conditions lower HCP levels to some extent, product yield was sacrificed. We obtained an optimal combination of high yield and low HCP content using an intermediate wash containing 0.5 M arginine (pH ≥ 8) or 2 M GuHCl (pH 8.5). That is likely to be a result of the chaotropic property of those reagents.
Outlook
Our results highlight the potential for improving protein A purification through the appropriate choice of intermediate wash buffer. We demonstrated that intermediate wash conditions can significantly affect purity levels in a final elution pool. An intermediate wash disrupts the interactions between HCPs and the resin as well as the HCP with a product of interest. Effective application of intermediate wash can improve efficiency of a protein A unit operation, which ultimately would influence product quality as well as productivity.
Acknowledgments
The authors acknowledge Gerd Kern and Sapna Mehtani for reviewing and contributing to this article.
References
1 Blank GS. Protein Purification. USPTO patent 6,797,814 B2 (28 September 2004).
2 Breece T, et al. Protein Purification. USPTO patent 6,870,034 B2 (22 March 2005).
3 Frauenschuh A, Bill K. Wash Solution and Method for Affinity Chromatography. World Intellectual Property Organization patent WO 2011/073389 (23 June 2011).
4 Shukla A, Hinckley P. Host-Cell Protein Clearance During Protein A Chromatography: Development of an Improved Column Wash Step. Biotechnol. Prog. 24(5) 2008: 1115–1121.
5 Sun S. Arginine Wash in Protein Purification Using Affinity Chromatography. World Intellectual Property Organization patent WO 2008/031020 A2 (13 March 2008) and USPTO patent 8,350,013 B2 (8 January 2013).
6 Sisodiya VN, et al. Studying Host-Cell Protein Interactions with Monoclonal Antibodies Using High-Throughput Protein A Chromatography. Biotechnol. J. 7(10) 2012: 1233–1241.
7 Kramarczyk JF. High-Throughput Screening of Chromatographic Resins and Excipients for Optimizing Selectivity. Biotechnology Engineering. Tufts University: Medford, MA, 2003.
8 Coffman JL, Kramarczyk JF, Kelley BD. High-Throughput Screening of Chromatographic Separations, 1: Method Development and Column Modeling. Biotechnol. Bioeng. 100(4) 2008: 605–618.
Corresponding author Melissa Holstein, PhD, ([email protected]) is an applications engineer, Kristen Cotoni is a scientist, and Nanying Bian, PhD, is a principal scientist at EMD Millipore, EMD Millipore Corporation, 80 Ashby Road, Bedford, MA 01730; www.emdmillipore.com.