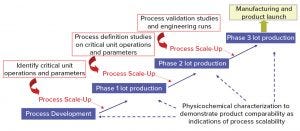
Figure 1: Vaccine process development – general approach
Scientists have made significant breakthroughs in bioprocess and analytical technologies for supporting vaccine development. Such technologies have helped vaccine manufacturers achieve consistent product purity and quality rapidly and cost effectively. Although interest in vaccine development and manufacture continues to increase because of the rapid growth of the global vaccine market, this area of the bioprocess industry remains challenging and complex.
Here we review the current constraints and complexities in the vaccine industry, specifically related to product development and manufacture. We describe the evolution of bioprocess and analytics innovations and technologies to overcome vaccine challenges, with an outlook into the future.
Challenges and Complexities
The global vaccine market has been growing rapidly and thus has been attracting interest and new players. The World Health Organization (WHO) (1) has reported that from 2000 to 2013, the vaccine market grew from 4 billion US dollars (USD) to 24 billion USD (Table 1). Market trends suggest that by 2028, the value of the vaccine market is estimated to be about 100 billion USD, growing at a compound annual growth rate (CAGR) of 11.02% (2, 3). And more than 120 new products are in development, 60 of which are of special importance for developing countries.
Vaccines are becoming a growth engine for the biopharmaceutical industry (1). Over the past few years, we have seen their status change within the industry as the number of mergers and acquisitions increases. A new business model for vaccines is emerging and attracting significant interest.
Although vaccines are biological products derived from living organisms, they are more complex than many traditional therapeutics, both in terms of their components and the technologies required to produce them. Vaccines typically are administered to healthy individuals (prophylaxis), whereas other therapies are given to persons with medical conditions. Vaccines also are more difficult to develop and manufacture than many other biologics and thus are more difficult to make in “generic” form. So vaccine products are more likely to retain their commercial value (4).
Vaccines are manufactured using a wide range of cell substrates (e.g., mammalian, insect, microbial, and fungal cell lines). New antigens also typically require novel cell substrates. Table 2 lists the number of different cell substrates used in vaccine development and manufacture. The list includes a diversity of vaccine products, including live attenuated vaccines, inactivated or detoxified vaccines, subunit vaccines, polysaccharides, virus-like particles, and protein complexes. Each vaccine type has its own degree of complexity and range of biochemical and biological properties.
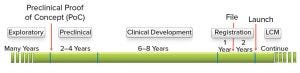
Figure 2: Typical timelines for vaccine development (9) (LCM = lifecycle management)
Figure 1 shows a general approach for vaccine process development for drug substance. Process development starts at a laboratory scale for identification of unit operations and parameters, followed by step-wise scale-up (usually in a 20-L fermentation or cell culture) for production of good manufacturing practice (GMP) materials for phase 1 clinical studies. Process definition studies are performed on critical unit operations and parameters using a design of experiments (DoE) before scaling up to 200 L (normally for phase 2). Before phase 3 (~2,000-L scale), process validation and engineering runs are required. During process development and scale-up, scientists perform biophysical, chemical, and biological characterization to acquire product and process knowledge to support and demonstrate product comparability and process scalability.
In addition to the above complexities of development, vaccine manufacturers face a high-cost and high-risk business environment, competition with other major vaccine manufacturers, increasing compliance and safety expectations, and highly sophisticated technology-driven platforms. Developing and licensing a vaccine product typically takes 12–14 years (Figure 2). Total costs can exceed $1 billion per new development, and the overall success rate from early phase development to license was <10% during 2000–2010 (3–5). A 2016 study showed a ~20% success rate from phase 1 to license (Table 3) (7).
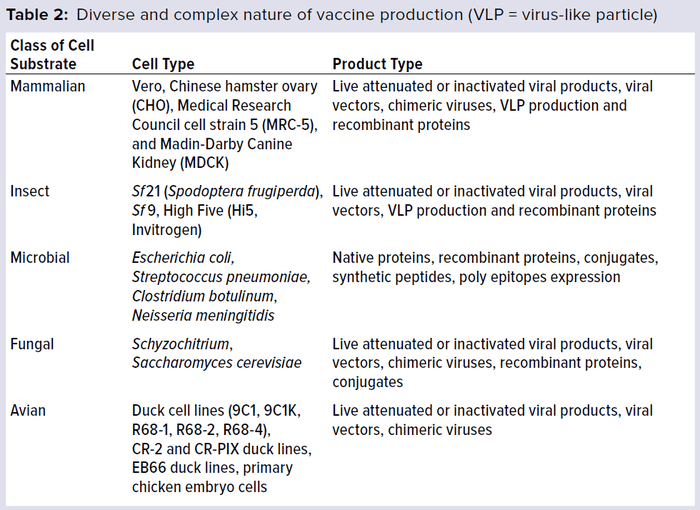
A Changing Market
The number of vaccines entering the market has been driven by technology advancement and availability. For example, from the mid-1800s to mid-20th century, only a small number of vaccines based on fermentation and genetics entered the market. Those numbers rose significantly during the second half of the 20th century, with new vaccines entering the market. Development of novel vaccines was driven by immunology research advancing to the point at which it could distinguish between protection mediated by antibodies and that mediated by cells (lymphocytes). Vaccine innovations also advanced when passage in cell culture permitted selection of attenuated mutants and implementation of technologies that enabled purification of proteins, polysaccharides, conjugation, and genetically engineering biological components (5–8).
Since the 1980s, the booming bioindustry has stimulated an evolution of biopharmaceutical technology to address the needs of high yields, high productivity, and increased speed and efficiency. In return, the rapid advancement of bioprocess technology has further fueled the growth of the biopharmaceutical industry, including vaccines. Over $800 million is spent in nonclinical investment per vaccine development (5).
The vaccine industry is challenged to develop high-quality products at decreased cost and within shortened timelines. The need to reach the market first highlights the importance for fast process development strategies and techniques. Such pressures have driven the vaccine industry to embrace innovative technologies. In return, shortening process development times will accelerate overall vaccine product development timelines and rapidly deliver safe and high-quality products to a global market.
Single-Use Technologies and Closed Systems
Some vaccine manufacturers face additional difficulties. These include the need to work with small batch sizes and varied product portfolios. Pandemic outbreaks that require rapid responses from vaccine developers and use of highly potent ingredients place large demands on cleaning processes. Given such pressures, single-use fill–finish assemblies can be an attractive option for vaccine manufacturing facilities (9, 10).
The biologics industry has been using single-use technologies for over 10 years to the point at which it can be considered mainstream in bioprocessing, including in vaccine development and manufacturing (8, 11). Key advancements include single-use bioreactors to disposable products for final formulation and filling. A number of vendors provide single-use bioreactors, centrifuges, depth filters, column chromatography systems, and bags for media preparation and final formulation (8).
A New Closed System with Single-Use Technologies: Some single-use systems have been established in process development and are making their way into manufacturing. For example, our company has developed a closed, single-use process system (from bench top to 100-L scale process) for a serum-free, live viral vaccine product, with close collaboration between suppliers and the vaccine industry (Figure 3). We have reproduced a robust purification process and significantly increased virus yield from 20–40 doses/L to 400–500 dose/L (a 10-fold increase). Furthermore, residual Vero DNA and host-cell proteins in this product were reduced significantly, and the purification process was shortened from three days to two days of operation. In addition, staff found the operations of the closed system much easier to manage than the previous system. Because it produces higher yields, the new system required fewer production runs. And the use of disposable technologies ensures that sterility is maintained throughout the process.
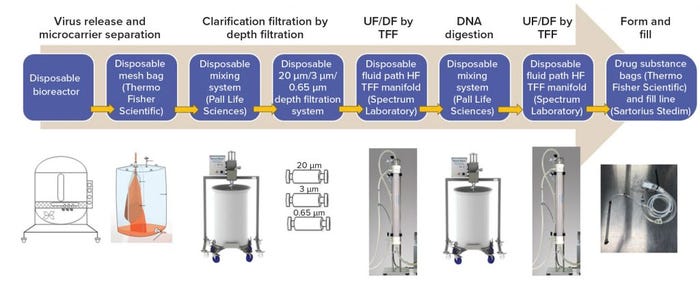
Figure 3: A completely closed system from manufacturing to formulation with single-use technologies (12); (UF = ultrafiltration, DF = diafiltration, TFF = tangential-flow filtration, HF = hollow fiber)
The net result was an overall reduction in development time and manufacturing costs. Facility turnover was easier and faster with this than with the previous system, and validation of fixed assets such as stainless steel fermentors, tanks, and centrifuges was no longer necessary. We completed multiple demonstrations and GMP runs with single-use technologies in a closed system, and all products passed sterility testing. Application of single-use technologies provided opportunities to reduce fixed costs, amount of equipment, and cleaning validation while increasing facility and process flexibility and accelerating process development time. Disposables play a key role in addressing the industrial challenges associated with developing high quality processes while driving down operational costs.
The closed system demonstrates the benefit of applying disposable technology to accelerate process development. Implementation of such technologies is anticipated to reduce process development time, trim manufacturing costs, and increase process and facility flexibility, thus facilitating expansion of manufacturing activities and increasing process development efficiency.
High-Throughput Technology to Accelerate Process Development
Our company also has been developing scale-down models and high-throughput technologies to accelerate and reduce process development costs. Figure 4 shows the effects of mathematical modeling and development of a scale-down model for one of the company’s products to solve scale-up and troubleshooting challenges. The data demonstrate successful studies at the 2-L scale that can be applied at the 200-L scale. Over the years, we have introduced a number of high-throughput process and analytical equipment systems to accelerate product development, reduce cost, and increase overall understanding of processes and products.
Table 4 lists comparable results obtained between the conventional approach and high-throughput system for vaccine process development from upstream microbial and cell culture to downstream purification and to product formulation. Using the high-throughput approach led to significant savings in time, resources, and material (11, 12). High-throughput process development will be successful only when high-throughput in-process test capabilities are established efficiently. That is the next area in vaccine development to address.
Examples presented above demonstrate the benefit of applying high-throughput technology to accelerate process development. The implementation of such technologies is anticipated to reduce process development time, thus accelerating clinical entry for a wider range of innovative vaccine candidates.
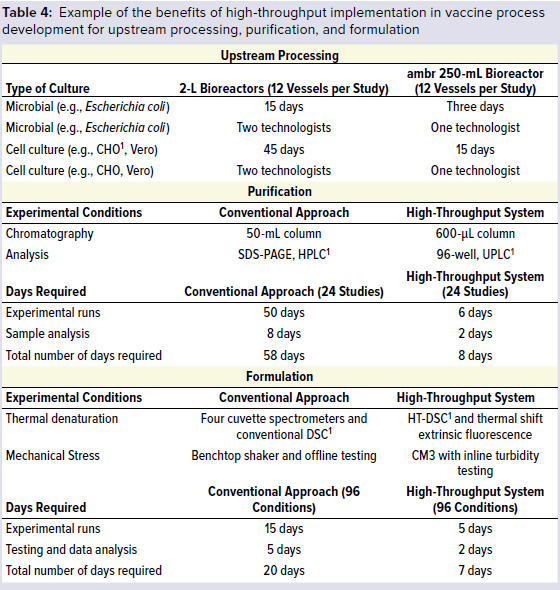
1 CHO = Chinese hamster ovary, SDS-PAGE = sodium dodecyl sulfate polyacrylamide gel electrophoresis, HPLC = high-performance liquid chromatography, UPLC = ultraperformance liquid chromatography, DSC = differential scanning calorimeter, high-temperature differential calorimetry
Continuous Manufacturing
Ideally, continuous manufacturing includes processes that are integrated, based on a systems approach, have model-based control, and make use of continuous flow. A continuous manufacturing process is designed as a whole, so distinctions between upstream and downstream or between drug substance and drug product would ultimately disappear.
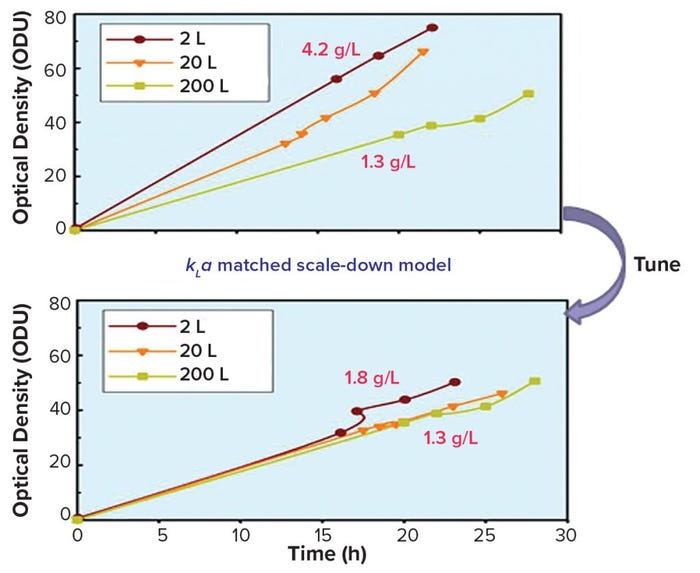
Figure 4: Mathematical modeling and scale-down model to solve scale-up challenges
Over the past decade, biomanufacturers have made significant investments in continuous manufacturing development (13) because it holds great promise to reduce process steps, footprint, and equipment size while increasing product quality and cost savings (14–16). Although the use of continuous processing still is in its early stages, the vaccine industry has embraced it and is ready to explore the full advantages associated with this approach. Many technical challenges remain for implementing a continuous process in vaccine manufacturing, including the need to develop continuous steps for viral inactivation and removal, continuous ultrafiltration and diafiltration, and in-process analytical tools for monitoring and controlling an entire process.
Continuous perfusion processes (upstream) are not new. Perfusion cell culture has been used extensively by the biotechnology community because it has enabled production of unstable proteins, which is difficult or impossible to accomplish with traditional fed-batch technologies. Relatively speaking, development of continuous downstream purification is lagging in the field, but that is about to change. In recent years, an increasing number of technologies have become available to support continuous downstream processing (Figure 5).
According to Janet Woodcock (director of the Center for Drug Evaluation and Research, CDER, FDA), one difficulty in implementing continuous biopharmaceutical manufacturing is developing advanced control strategies and incorporating real-time data strategies for critical quality attributes (CQAs). Other challenges include effectively integrating downstream unit operations to satisfy purity requirements as well as reaching bioprocess community agreement on real-time release methods (13).
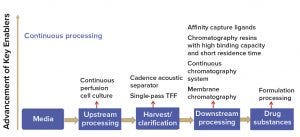
Figure 5: Rapid technology advancement of key enablers for continuous manufacturing (TFF = tangential-flow filtration)
Biomanufacturers (including vaccine developers) increasingly have been accepting quality by design (QbD) principles. QbD emphasizes process understanding and process control, which also are essential to continuous processing, especially the application of process control strategies as appropriate analytical tools for successful continuous manufacturing (Figure 6).
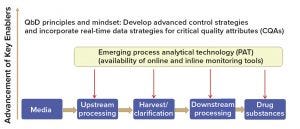
Figure 6: Building the foundation for continuous manufacturing using quality by design (QbD) principles and mindset
The biopharmaceutical industry’s cautious approach to continuous manufacturing should not be surprising. The industry’s aversion to risk relates to the large percentage of revenues invested in research and development, the high rate of failure at every stage of product development, and the years needed to bring a product to market (17).
Analytical Innovations
“Cutting-edge” analytical technologies accelerate product development and enable improved understanding of products and processes. This helps manufacturers to quickly troubleshoot problems with their processes. Technologies highlighted here are mass spectrometry (MS), capillary electrophoresis (CE), and high-throughput sequencing (HTS) because they have improved the bioindustry’s ability to address issues rapidly and understand its products.
Advantages of using MS technology include its high sensitivity, selectivity, and accuracy. It also can be used as an in-line detection method such as with high-performance liquid chromatography (HPLC) or ultraperformance liquid chromatography (UPLC) to provide highly sensitive, multidimensional analysis of complex samples. That in turn enables accurate and robust monitoring and control of key quality attributes of vaccines (e.g., content and identity) and facilitates fast and reliable decision-making during product development.
MS can be used to monitor parameters such as protein antigen content, intact mass, identity, and posttranslational modifications in a single analytical workflow, thus enabling the introduction of multiattribute monitoring (MAM) of processes using a single instrument or technology. Our company has successfully applied MS methods to identify and quantitate small-molecule impurities, excipients, and adjuvants, with sensitivities in the femtomolar range.
Liquid chromatography mass spectrometry (LC–MS) and LC–MS/MS are used routinely for highly accurate determination of protein intact mass and amino acid sequences to identify target proteins, HCP impurities, and truncations or other covalent modifications. Advanced instrument and sample-preparation methodologies have enabled identification of multiple protein antigens in adjuvanted combination vaccines for product release in a single assay workflow, thus replacing multiple immunochemical assays at a fraction of the cost. Hydrogen–deuterium exchange (HDX) MS is used for epitope mapping of neutralizing monoclonal antibodies (MAbs) used as critical reagents in immunoassays. Future applications currently being explored include quantitative MS as a single technology to assess antigen content (as an early surrogate of vaccine potency) at multiple product stages and expansion of in-house capabilities beyond LC–MS through acquisition of CE–MS instrumentation and development of supporting workflows and methods.
CE technology has been instrumental for purity and quantitation analyses of vaccine antigen proteins, quantitative stability-indicating assays for vaccine antigen proteins, and as a replacement for older quantitative methods such as sodium dodecyl sulfate polyacrylamide gel electrophoresis (SDS-PAGE) as the platform of choice for purity assays for new investigational target protein antigens. Key benefits of using CE include high throughput and resolution as well as potential for automation of both sample preparation and data analysis. Future opportunities include interfacing of CE with MS systems (CE–MS) for highly selective and sensitive online detection.
HTS technology platform is an alternative to in vivo (animal) and in vitro viral adventitious agents testing (18). Development of this technology was in response to the need to prevent a potential porcine circovirus-like crisis that occurred in the industry when HTS technology detected a broader range of adventitious agents (18). In 2011, our company began exploring different HTS technologies and started the development of an automated analysis pipeline. HTS technology allows prescreening of cell substrates and viral seed preparations rapidly and with sensitivity to reduce risk of contamination before investing in process development.
Our company also is developing HTS technology to streamline an adventitious agent testing package with the replacement of in vivo and in vitro adventitious agent tests (19). HTS has been beneficial during investigation for adventitious agents. Our company has been able to identify a viral isolate that caused a cytopathic effect during testing for the absence of active virus, to identify a viral positive control as a potential cause for an atypical monolayer result, to determine Acinetobacter as the most probable cause for the embryo deaths observed during egg testing of an extended cell bank, and to identify a novel mammalian orthoreovirus type 1 as the cause of the atypical result observed in a primary monkey kidney control cell lot (19).
Prepared for the Future
The speed of vaccine development and the number of vaccines on the market have been driven mainly by the advancement and implementation of innovative technologies. Our company has seen significant pay-back, both in time and cost, resulting from the implementation of single-use technologies, high-throughput screening systems, and cutting-edge analytical methods. On the horizon, a number of novel technologies making their way into vaccine development and manufacturing will continue to shape the way operators perform their work and drive the overall industry to greater heights. Such technologies include continuous manufacturing, transformative technologies such as mRNA vaccine platforms, data mining and artificial intelligence, molecular imprinting, novel adjuvants, immunomodulation, and novel expression systems. As Woodcock pointed out in a recent workshop, 20 years from now biological product manufacturing is unlikely to look like it does today. The field will advance, and the outlines of where the field should go already are visible (13). By embracing technology innovation and taking incremental steps and calculated risks, the vaccine industry will be well prepared for the future.
References
1 Kaddar M. Global Vaccine Market Features and Trends. WHO — Immunization, Vaccines, and Biologicals (IVB): Geneva, Switzerland, 2012.
2 Global Vaccines Market Forecasts 2018–2025: Strong Pipeline, Rising Adoption of Vaccination, Increasing Government Focus on Immunization Programs and Technological Progress in Vaccine Administration. PR Newswire, 12 December 2018.
3 Global Vaccine Market Analysis and Forecast (2018–2028): A $103.57 Billion Opportunity, Growing at a CAGR of 11.02%. Globe Newswire, Research and Markets: Dublin, Ireland, 10 December 2018.
4 Principles of Vaccination. Centers for Disease Control and Prevention: Atlanta, GA, 11 May 2010.
5 Plotkin S, et al. The Complexity and Cost of Vaccine Manufacturing: An Overview. Vaccine 35(33) 2017: 4064–4071; doi:10.1016/j.vaccine.2017.06.003.
6 Woppmann A. Strategies and Challenges in Product Development from the Preclinical Stage to Commercialization. BIO International Convention, Toronto, ON, Canada, 2002.
7 Clinical Development Success Rates 2006–2015. BIO Industry Analysis, Biomedtracker, Amplion, 2016.
8 General Metrics Study. KMR Group, R&D: Chicago, IL, 2011.
9 D’Amore T. Yang Y-P. Increasing Flexibility in Vaccine Manufacturing Processes and Facilities with Single-Use Disposable Technologies. BioPharma Asia July/August 2013: 8–17.
10 Jenness E, Walker S. Advantages of Single-Use Technology for Vaccine Fill–Finish Operations. PDA J. Pharm. Sci. Technol. 68(4) 2014: 381–383; doi:10.5731/pdajpst. 2014.00985.
11 Yang Y-P. Advances in Purification Technologies Accelerate Vaccine Development. Am. Pharm. Rev. 19(5) 2016: 52–59.
12 Yang Y-P. From Purification to Vaccine Development: A Challenging Yet Rewarding Journey. BioPharma Asia September/October 2017: 28–34.
13 DePalma A. Continuous Bioprocessing: Upstream, Downstream, Ready for Prime Time? BioProcess Int. 14(5) 2016: insert.
14 Continuous Manufacturing for the Modernization of Pharmaceutical Production: Proceedings of a Workshop. National Academies Press (US): Washington, DC, 30 January 2019.
15 Konstantinov K, Cooney C. White Papers from International Symposium on Continuous Manufacturing of Pharmaceuticals. Continuous Manufacturing Symposium, MIT, May 2014; doi: 10.1002/jps.24268.
16 Zydney AL. Perspectives on Integrated Continuous Bioprocessing: Opportunities and Challenges. Curr. Op. Chem. Eng. 10, 2015: 8–13; doi: 10.1016/j.coche.2015.07.005.
17 Jacoby R, et al. Advanced Biopharmaceutical Manufacturing: An Evolution Underway. Deloitte Development, LLC: Stamford, CT, 2015.
18 Charlebois RL, et al. Cataloguing the Taxonomic Origins of Sequences from a Heterogeneous Sample Using Phylogenomics: Applications in Adventitious Agent Detection. PDA J. Pharm. Sci. Technol. 68(6) 2014: 602–618; doi:10.5731/pdajpst.2014.01023.
19 Ng SH, et. al. Preliminary Evaluation of Next-Generation Sequencing Performance Relative to qPCR and In Vitro Cell Culture Tests for Human Cytomegalovirus. PDA J. Pharm. Sci. Technol. 68(6) 2014: 563–571; doi:10.5731/pdajpst.2014.01013. c
Corresponding author Tony D’Amore is vice president and global head of product research and development, and Yan-ping Yang is associate vice president and head of bioprocess research and development in North America at Sanofi Pasteur, 1755 Steeles Avenue West, Toronto, Ontario, Canada M2R 3T4; 1-416-667-2745; [email protected].