Bispecific antibodies (bsAbs) are genetically engineered recombinant proteins with two distinct domains binding to two antigens or epitopes simultaneously (1). The concept for bsAbs was proposed by Nisonoff and Rivers in 1961 and later confirmed by Fudenberg et al. in 1964 (2, 3). Great progress has been made for bsAb development over the past few decades with the advancements of genetic engineering approaches, protein modeling and simulation technologies, and manufacturing processes and experience (4–8). As Table 1 shows, bsAbs demonstrate versatile mechanisms of actions (MoAs) such as cell bridging (e.g., mosunetuzumab-axgb), blood coagulation factor-bridging (e.g., emicizumab-kxwh), receptor-activating or -blocking (e.g., ozoralizumab and faricimab-svoa), receptor or ligand internalization or clustering, cofactor mimicking (e.g., emicizumab-kxwh), and retargeting of cytotoxic effector cells (7).
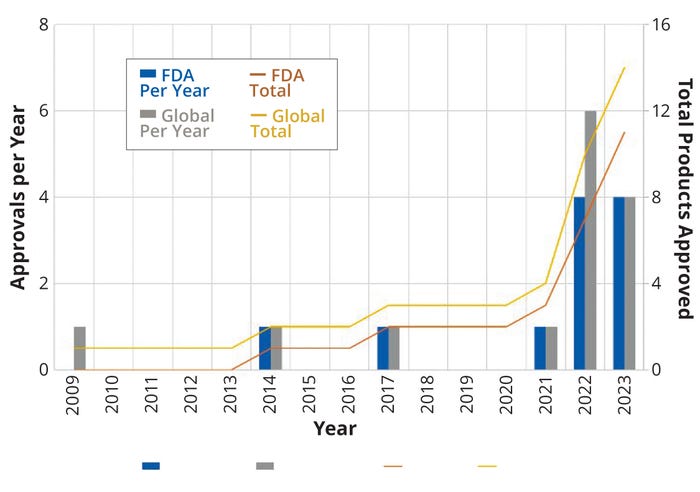
Figure 1: Regulatory approvals per year (left axis) and trend lines (right axis) for bispecific antibodies (bsAB) in the United States and around the world.
By the end of 2023, 14 bsAbs were approved by global regulatory authorities (Table 1, Figure 1). Most are cancer immunotherapies, with a few marketed for treating chronic inflammatory and hematologic disorders (Figure 2). So far, the approved bsAb cancer immunotherapies target either two immunomodulators on T cells or other targets on endothelial cells or cluster-of-differentiation 3 (CD3) proteins on T cells and tumor antigens on cancerous/malignant cells. Examples of the latter targets include epithelial cell adhesion molecule (EpCAM), CD19 or CD20, B-cell maturation antigen (BCMA), G-protein–coupled receptor, class C 5D (GPCR5D), and glycoprotein 100 (gp100).
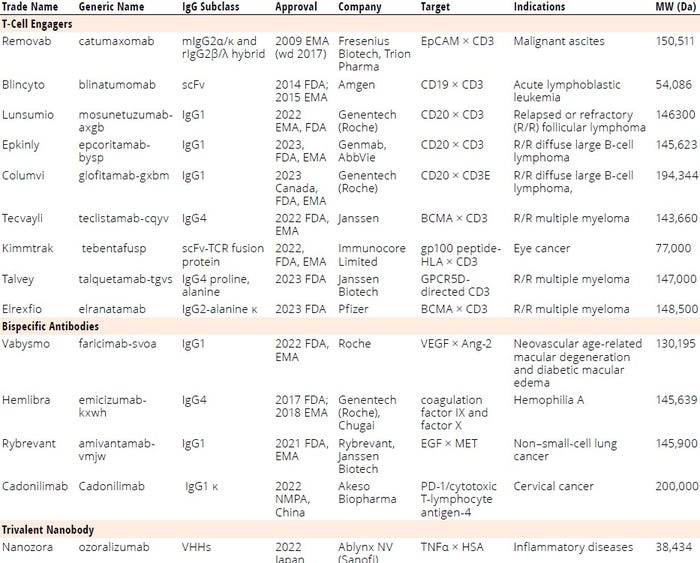
Table 1: List of regulatory-approved bi-/multispecific antibodies; BCMA = B-cell maturation antigen, CD = cluster of differentiation (protein), EMA = European Medicines Agency, EpCAM = epithelial cell adhesion molecule, FDA = US Food and Drug Administration, gp100 = glycoprotein 100, GPCR = G-protein–coupled receptor, HLA = human leukocyte antigen, IgG = immunoglobulin G, scFv = single-chain variable fragment, VEGF = vascular endothelial growth factor, VHH = variable heavy chain (fragment), wd = withdrawn.
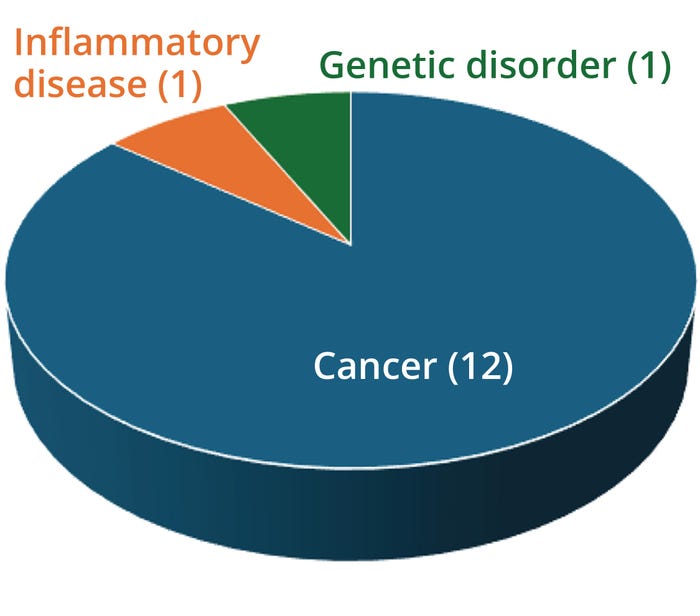
Figure 2: Applications of 14 approved bispecific antibodies (bsAbs).
T-cell engagers (TCEs) redirect cytotoxic T cells to kill cancer cells, activate T cells through CD3 agonism, and form potent cytolytic synapses between T cells and cancer cells, thus mounting a cytolytic antitumor response (9). Nine of the approved bsAbs are TCEs targeting
• CD3/CD19 (blinatumomab)
• CD3/CD20 for relapsed or refractory (R/R) follicular lymphoma (mosunetuzumab-axgb)
• CD3/CD20 for R/R diffuse large B-cell lymphoma (e.g., epcoritamab-bysp and glofitamab-gxbm)
• CD3/BCMA for R/R multiple myeloma (teclistamab-cqyv and elranatamab-bcmm)
• CD3/gp100 peptide–human leukocyte antigen (HLA) for eye cancer (tebentafusp-tebn)
• CD3/EpCAM for malignant ascites (catumaxomab)
• CD3/GPRC5D for R/R multiple myeloma (talquetamab-tgvs).
Currently, more than 200 bsAbs are in clinical trials for radioimmunotherapy, antiviral infection treatment (e.g., Ebola), neurotherapy (e.g., Alzheimer’s disease), and cancer therapy (for solid tumors) (6, 10–12). Developing biologic products from discovery to commercialization is a long journey with high costs and low rates of regulatory approval (Figure 3). For example, Hemlibra (emicizumab-kxwh) for hemophilia A treatment took over 15 years to achieve market approval (13).
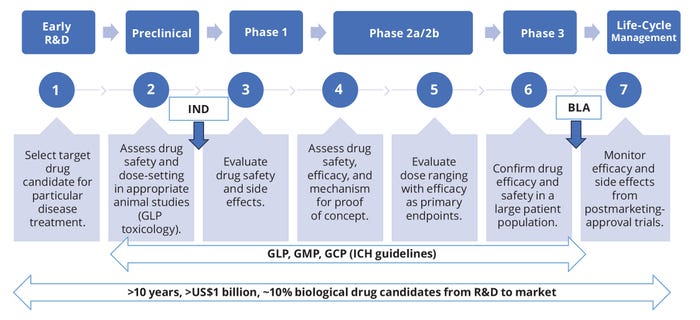
Figure 3: Road map of biologic drug research and development (R&D); BLA = biologics license application, GCP/GLP/GMP = good clinical/laboratory/manufacturing practice, ICH = International Council on Harmonisation, IND = investigational new drug (application).
The first step in product development is to select the best candidate based on a molecule’s physicochemical properties, format, target specificity, pharmacokinetics (PK), and intended therapeutic effects (Figure 3). Based on lessons learned from a number of bsAb commercialization projects, we highlight herein some chemistry, manufacturing, and controls (CMC) considerations based on bsAb physiochemical properties. We highlight quality and regulatory requirements as well as concerns that can arise in developing assay methods, manufacturing processes, and formulations. Our purpose is to apply these learnings to future bsAb product-development programs.
Considerations from bsAb Physiochemical Properties
Key physiochemical properties of bsAbs include molecular size, protein sequence, secondary and tertiary structures, hydrophobicity, charge profile, posttranslational modifications (PTMs, e.g., glycosylation, cyclization, deamidation, oxidation, and clipping), isoelectric point (pI), and structural format. So far, >100 bsAb structural formats and >30 technology platforms have been reported (4, 6). Selection of a structural format — e.g., immunoglobulin (IgG) or antibody-fragment format — depends on a given molecule’s therapeutic purpose, MoA (Table 1), functionality, and developability. Eleven commercial bsAbs are IgG based (e.g., IgG1, IgG2, IgG4), and seven are IgG TCEs. IgG-based bsAbs have a similar crystallizable fragment (Fc) structure to that of native/wild-type human antibodies. Fc-mediated effector functions are associated with antibody-dependent cell-mediated cytotoxicity (ADCC), complement-dependent cytotoxicity (CDC), and antibody-dependent cell phagocytosis (ADCP).
Fc regions can enhance antibody half-life, stability, and solubility while facilitating purification by protein A affinity chromatography (1, 8, 14). However, IgG-based bsAbs have disadvantages related to expression levels and yields, aggregation, and the presence of homodimers and other by-products (15, 16). Efforts to minimize impurities and enhance product manufacturability have led to several structural variations (e.g., knob-into-hole and Roche’s CrossMab technology) (17). To minimize Fc-mediated effects, some developers have adopted Fc silencing (e.g., mosunetuzumab) (18) or antibody fragments (e.g., blinatumomab).
Advantages of the three approved fragment-based bsAbs over Fc-containing bispecifics include their smaller size, less complex structures, increased tissue-penetration ability, and conformational flexibilities (7). And in process development, fragment-based bsAbs are easily purified and manufactured with higher yields (7). However, the lack of an Fc domain can bring some molecular instability and reduce serum half-life (19). Strategies for overcoming such issues include lyophilization (e.g., blinatumomab) for stability and engineering in a human serum albumin (HSA) binding domain for extended half-life (e.g., ozoralizumab).
Considerations from Process Development
Process development and CMC activities usually begin once a bsAb candidate has been selected from discovery research (Figure 4). This work encompasses cell-line development (CLD), cell-culture process development (CCPD), downstream process development (DSPD), analytical method development (AMD), formulation and stability studies, support of drug-substance (DS) manufacturing, and drug-product (DP) fill–finish.
Figure 4: Key considerations for bispecific antibodies (bsAb) development; SQIPE = safety, quality, identity, purity, and efficacy.
ELISA = enzyme-linked immunosorbent assay, HVAC = heating, ventilation, and air conditioning, LC-MS = liquid chromatography with mass spectrometry, pI = isoelectric point,
SEC-HPLC = size-exclusion high-performance liquid chromatography, SDS-PAGE = sodium dodecyl sulfate–polyacrylamide gel electrophoresis.
Considerations from CLD and CCPD: CLD includes selection of a host cell line, optimization of gene codons and signal peptides, design of genetic vectors (e.g., origins of replication, antibiotic resistance genes, promoters, and enhancers), transfection of genes (e.g., using polyethylenimine or liposomes) and selection of clones (20). Cell lines derived from Chinese hamster ovary (CHO) cells, including CHO-K1 and CHO-GS, are used widely for expressing mAbs and IgG-based bsAbs because of the cells’ tolerance to manufacturing processes, rapid growth rates, and ability to perform human-compatible PTMs (8). However, genetic instability of CHO cells can lead to declining growth rates, expression titers, and product quality due to chromosome rearrangement and PTMs (21).
Clone selection is based on cell-growth profiles, which describe expression titers, viability, viable cell density (VCD), metabolism, monoclonality, gene copy number, genotype and phenotype stability, product quality (percentage of monomers, aggregates, fragments, homodimers, mispaired products, and glyco- and charge variants), binding potency (determined by immunoassays or cell-based assays) and biosafety (endotoxin, bioburden, and viruses). Glycosylation is a critical quality attribute (CQA) that influences drug absorption, serum half-life, immunogenicity, and clearance from serum (22). Small-chain variable fragment (scFv)–based bsAbs (e.g., blinatumomab and tebentafusp) can be produced either by CHO cells or Escherichia coli bacteria.
Once a top clone has been selected, a research cell bank (RCB) is generated for media selection, feed strategy development, and process-parameter determination for CCPD. The RCB is used to create both a master cell bank (MCB) and an initial working cell bank (WCB) for large-scale manufacturing after host-cell characterization and stability and biosafety testing (23). Key process parameters (KPPs) and critical process parameters (CPPs) usually are determined for measuring and monitoring process performance and product CQAs (e.g., glycans, charge, and safety), respectively, from CLD through CCPD and DSPD (24). For example, sialylation or sialic-acid content can affect product function and stability (25). From a CCPD perspective, low glucose concentration (<0.7 mM) in media has decreased sialyation and increased mannose (26). Sialic acid content also can be enhanced with media additives (e.g., galactose, glucosamine, N-acetylmannosamine dexamethasone, and manganese) and/or cell-culture process controls such as maintaining high dissolved oxygen (DO) and proper glucose feeding to lower lactate accumulation and mannose synthesis (27, 28).
Raw-material components of animal origin should be tested (e.g., for prions) and eliminated from use in media and buffers for good manufacturing practice (GMP) operations. Human carcinogenic components such as nitrosamines also should be tested and eliminated from use (29). Compendial grades of raw materials should comply with the pharmacopeial requirements of a product’s intended market. Materials should be purchased from qualified suppliers with certificates of analysis (CoAs). Noncompendial materials, if needed, should be purchased from approved suppliers and tested against defined sets of acceptance criteria for clinical and/or commercial products.
Considerations or DSPD Process Design, Development, and Scale-Up: A bsAb separation/purification process should be efficient, scalable, robust, repeatable, and controllable, providing yields as high as possible (17, 30). Purified products should meet predetermined product safety, quality, identity, purity, and efficacy (SQIPE) requirements (14). For IgG-based bsAbs, the familiar mAb purification platform approach is effective for early stage clinical trial support — saving time, resources, and cost. The platform consists of affinity capture of product followed by low-pH or detergent treatment for retrovirus inactivation, ion-exchange and/or mixed-mode chromatography for impurity and virus removal, viral filtration (VF) for small-virus clearance, tangential-flow filtration (TFF) for DS formulation, and 0.22-µm filtration for bioburden control (14, 17).
Intermediate and polishing chromatography steps play vital roles for removal of process- and product-related impurities. For example, mixed-mode products such as Cytiva’s CaptoMMC ImpRes resin and cation exchangers such as Cytiva’s Capto S ImpAct resin can remove product-related impurities (e.g., aggregates and fragments) and process-related impurities (e.g., host-cell proteins, HCPs) effectively. Alternative purification approaches must be designed and developed for purification of fragment-based bsAbs. One example is light-chain affinity capture (17).
To ensure process consistency, yield, and product SQIPE, chromatography column quality — e.g., asymmetry and height equivalent to a theoretical plate (HETP) — should be controlled throughout process scaling. High loading capacities and reuse of resins can help to reduce costs related to raw materials in chromatographic unit operations. Additionally, minimizing system hold-up volumes from final ultrafiltration/diafiltration (UF/DF) and DS bulk filtration helps to limit product loss from lines, filters, and/or systems, particularly when DS concentration is high (>100 g/L).
Once a bsAb program enters late-stage clinical development, KPPs for process performance and CPPs for product SQIPE must be established from process characterization (PC) and process validation (PV) for inclusion in a biologics license application (BLA) or other regulatory submissions (14).
Analytical Considerations: AMD plays a key role in process development and manufacturing support. Analytical methods are used for physiochemical profile determination and process support, including in-process testing, formulation development, forced-degradation testing, DS/DP release and stability testing, reference-standard qualification, protein characterization, compatibility testing, and comparability testing. Developed methods play important roles in monitoring and controlling manufacturing process performance and product purity, aggregates, fragments, DS identity, molecular size and structure, charge variants, glycosylation, stability, safety, and potency (7).
In early stage product development, platform methods such as commercial enzyme-linked immunosorbent assay (ELISA) kits (e.g., from Cygnus Technologies) are acceptable for monitoring impurities such as HCPs. Product-specific methods typically are qualified and validated for BLA filing support. To evaluate the multiple-binding properties of bsAbs, two or more binding ELISA methods usually are developed from early- to later stages. Cell-based assays are required for BLA support (7).
AMD is important for CQA evaluations during process qualification and validation. CQAs can be associated with product properties (e.g., primary structure), MoAs (e.g., T-cell activation or antigen binding), charge variants (e.g., acidic or basic), PTMs (e.g., glycosylation, deamidation, and oxidation), impurities (e.g., aggregates, fragments, HCPs and host-cell DNA, and residual protein A), and adventitious agents (e.g., bioburden, endotoxin, and viruses). Aggregates and/or fragments usually become CQAs because they can compromise product safety and efficacy. Commonly used methods for release and stability acceptance criteria or setting specifications for aggregates and fragments control include reduced/nonreduced sodium dodecyl sulfate–polyacrylamide gel electrophoresis (SDS-PAGE) or capillary electrophoresis (CE-SDS) and high-performance liquid chromatography (HPLC) using size-exclusion (SEC-HPLC) or reversed-phase (RP-HPLC) media.
Endotoxins need to be well-controlled to prevent immunogenicity in patients (31). CQA specification setting may be based on clinical data, product-specific knowledge, applicant’s experience, formulation development studies, storage data, process effects, and/or manufacturing experience.
Considerations of Formulation and Stability: Formulation studies for bsAbs evaluate a number of DP ingredients, characteristics, and operations:
• DS integrity (sequence, structure, aggregation, degradation, folding, and assembly), potency, and functionality
• buffers (e.g., acetate, citrate, histidine, and phosphate)
• pH, protein concentration, viscosity, osmolality, temperature, humidity, and light exposure
• excipients (e.g., surfactants, amino acids, and sugars)
• containers and closures (e.g., vials, syringes, or bags)
• formulation operations (e.g., shear forces and freeze–thaw cycles).
Such testing evaluates changes associated with buffer pH and excipients, often including short-term “accelerated” stability studies for assessing osmolality and charge profiles; protein concentration, and surfactant effects; DP appearance and the presence or absence of visible/subvisible particles; and percentages of monomers, aggregates, and fragments.
Developing formulation conditions for bsAbs can be challenging because of their heterogeneity in N-terminal cyclization (e.g., Gln, Glu), C-terminal lysine cleavage, glycosylation, glycation, sialylation, deamidation (e.g., Asp, Gln), and oxidation (e.g., Met). Glycosylation-related heterogeneity influences drug activity, safety, efficacy, PK, and stability. Table 2 provides formulation ingredients, pH, protein concentrations, and forms (e.g., lyophilization, liquid) of the marketed bsAb products listed in Table 1. Such data can provide starting points to help developers of new bsAb formulation screening methods and save time, resources, and costs. Formulators also consider prior knowledge related to mAbs and other protein DPs as another good starting place, with a focus on using only excipients on the US Food and Drug Administration’s (FDA’s) “Generally Recognized as Safe” (GRaS) list, deviating from those only when absolutely necessary.
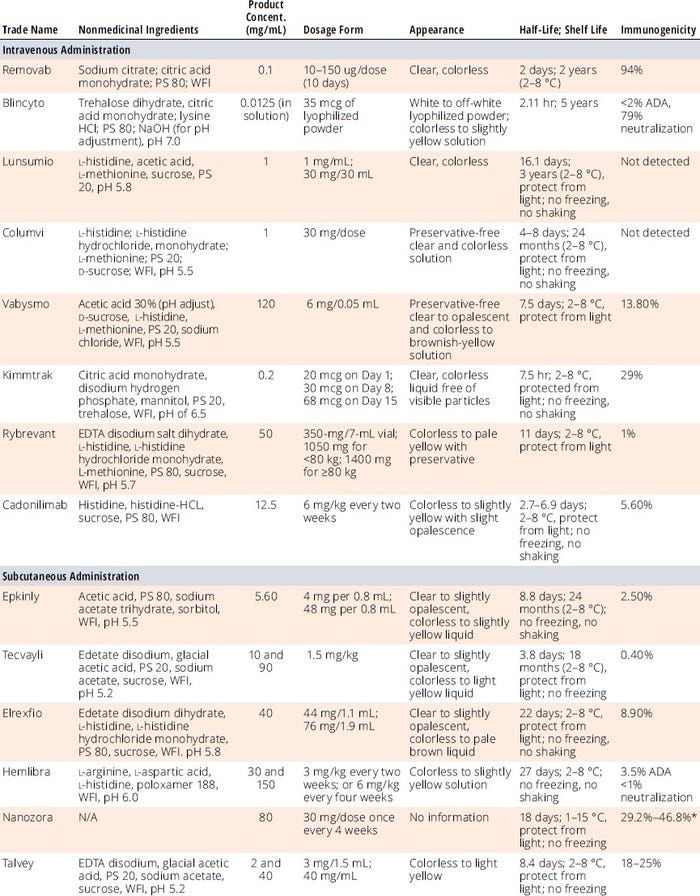
Table 2: Formulation, dosage, and stability information for regulatory-approved bi-/multispecific antibody drug products.
ADA = antidrug antibodies, EDTA = ethylenediaminetetraacetic acid, PS = polysorbate, WFI = water for injection
* Takeuchi T. Structural, Nonclinical, and Clinical Features of Ozoralizumab: A Novel Tumour Necrosis Factor Inhibitor. Mod. Rheumatol. 33(6) 2023: 1059–1067;
https://doi.org/10.1093/mr/road038.
Regarding DS and DP stability studies, it is generally acceptable to submit an investigational new drug (IND) application in the United States or an investigational medicinal product dossier (IMPD) in Europe for oncology therapies with one month of accelerated stability data, then supplement that information later as more data become available during clinical trials. The quality of preclinical DS batches should be representative of material used for clinical studies. For late-stage development, at least three batches with six months of stability data at minimum are required in BLA and equivalent regulatory submissions (32). For DPs, the minimum six-month stability should be determined case by case (32). DP container quality for preclinical studies should be representative of what is used in clinical trials. Stability tests are conducted to measure DP strength, potency, purity, and characterization — including concentration, visual appearance, sterility, stabilizers, and container–closure integrity — under proper temperature, humidity, and light in accelerated and stress conditions.
Considerations for Scale-Up/-Down for Manufacturing Support: In CLD, cell-line phenotypic and genotypic stability usually are evaluated for 60–80 generations to support large-scale production (e.g., 10,000-L bioreactors) and as few as 45 generations for smaller scales (≤2000 L). For CCPD, the same media and process parameters should be applied across scales. Similar bioreactor geometry is highly recommended across scales; otherwise, reliable scale-up/
-down models should be established. For purification, chromatography column bed heights should be maintained across scales along with residence time, load density, load ratio, peak cut, pH, conductivity, buffer composition, volumes for wash and elution, column packing quality, and so on. VF operations should use the same types of filters, load ratio (L/m2) and pressure (psi or bar), chase ratio, process-hold pause times between load and chase, and postuse integrity tests. The same principles should apply to TFF operations as well.
Considerations for Manufacturing: Key concerns in bispecifics DS manufacturing include raw material testing and release quality, equipment qualification and validation, facility design and qualification (especially closed systems), utility design and operation (e.g., water and heating ventilation and air conditioning systems, HVACs), environmental monitoring (e.g., nonviable particulates, airborne viable microbes, temperature, and humidity), process development (parameter targets and ranges), product quality and in-process stability, bioburden control, viral safety (with facility segregation from VF operations), good documentation practices (GDPs), and regulatory compliance. Current good manufacturing practice (CGMP) is the minimum requirement for manufacturing and controls (33). Each bispecific DS should meet predetermined acceptance criteria and specifications after release testing.
DP manufacturing procedures include aseptic filling (must be sterile and free from pyrogens and particles), product testing, labeling (written, printed, and graphic materials), packaging (cartons, receptacles, and wrappers, including all labeling), and storage conditions. Procedures should comply fully with 21 CFR Part 600 (33). For example, DP container quality for preclinical and clinical studies should be representative of that for commercial use. All final containers and closures must be clean and free of surface solids. DPs are tested for potency, purity, and characterization (e.g., visual appearance, sterility, stabilizers, and container–closures) under proper temperature, humidity, light, accelerated, and stress conditions. DP samples are retained for at least six months after their set expiration date unless a different time is specified in additional standards. They must be adequate quantities of representative materials from each lot of each product and sufficient for later examination and testing for safety and potency.
Considerations for Regulatory Compliance
Knowledge of regulatory guidelines and requirements — e.g., from the European Medicines Agency (EMA), US FDA, and World Health Organization (WHO) — is crucial throughout preclinical and clinical development to filing of market-authorization applications. Developers can meet with regulators along the way, and it helps to prepare phase-appropriate questionnaires for those meetings. Questions might relate to major changes in manufacturing sites, cell lines, media, purification column sequences, DS/DP release specifications, formulations, and stability/comparability studies during product development.
In 2021, WHO published a guideline for safe production and quality control of mAb development (20). It provides recommendations for control strategies (for raw materials, product quality, and biosafety), manufacturing (processes, filling, containers, records, labeling, reference preparation, and retained samples), testing (for purity, impurities, stability, and adventitious agents), and logistics (distribution and storage).
The International Council for Harmonisation of Technical Requirements for Pharmaceuticals for Human Use (ICH) published a helpful viral safety guideline in 2023 (34). Other documents — ICH E6(R2) on good clinical practice and 21 CFR Part 600 — address data integrity and record retention (33, 35). FDA and EMA published mAb manufacturing and test guidances in 1997 and 2016, respectively (29, 36). CMC regulations should embrace those same guidelines for bsAb development as well.
Lessons Learned from Commercial bsAb Products
IgG1- and IgG4-based molecules currently dominate the array of commercially available bsAb products (Table 1). Most marketed bsAbs so far are TCEs for cancer therapy. Fragment-based bsAbs also are used for cancer therapy and treatment of inflammatory conditions. The vast majority of commercial products are slightly acidic (pH 5.2–7.0) liquid formulations with bsAbs at relatively low concentration (<50 mg/mL) in a proper buffer matrix (typically citrate, acetate, histidine, or phosphate) with excipients such as sucrose, trehalose, methionine, polysorbate 80 or 20, and poloxamer 188. As with other biologics, method development for antidrug-antibody testing is optimized continuously from early clinical stages to postapproval improvement, as was the case with Vabysmo (faricimab-svoa).
CMC efforts for bsAb development, manufacturing, and clinical support should focus primarily on
• embracing regulatory guidelines
• engaging discovery and research groups as early as possible for bsAb developability and manufacturability assessment
• selecting the right molecular format for a candidate’s therapeutical purpose
• choosing the right gene expression system and cell line
• applying phase-appropriate strategies to process development and manufacturing
• improving product quality, safety, and efficacy continuously throughout a product’s life cycle.
References
1 Kontermann RE. Strategies To Extend Plasma Half-Lives of Recombinant Antibodies. Biodrugs 23(2) 2009: 93–109; https://doi.org/10.2165/00063030-200923020-00003.
2 Nisonoff A, Rivers MM. Recombination of a Mixture of Univalent Antibody Fragments of Different Specificity. Arch. Biochem. Biophys. 93(2) 1961: 460–462; https://doi.org/10.1016/0003-9861(61)90296-x.
3 Fudenberg HH, Drews G, Nisonoff A. Serologic Demonstration of Dual Specificity of Rabbit Bivalent Hybrid Antibody. J. Exp. Med. 119(1) 1964: 151–166; https://doi.org/10.1084/jem.119.1.151.
4 Spiess, C, Zhai Q, Carter PJ. Alternative Molecular Formats and Therapeutic Applications for Bispecific Antibodies. Mol. Immunol. 67(2) 2015: 95–106; https://doi.org/10.1016/j.molimm.2015.01.003.
5 Klein C, et al. Engineering Therapeutic Bispecific Antibodies Using CrossMab Technology. Methods 154, 2019: 21–31; https://doi.org/10.1016/j.ymeth.2018.11.008.
6 Ma J, et al. Bispecific Antibodies: From Research to Clinical Application. Front. Immunol. 12, 2021: 1–19; https://doi.org/10.3389/fimmu.2021.626616.
7 Register AC, Tarighat SS, Lee HY. Bioassay Development for Bispecific Antibodies: Challenges and Opportunities. Int. J. Mol. Sci. 22(10) 2021: 5350; https://doi.org/10.3390/ijms22105350.
8 Zhang T, Lin Y, Gao Q. Bispecific Antibodies Targeting Immunomodulatory Checkpoints for Cancer Therapy. Cancer Biol. Med. 20(3) 2023: 181–195; https://doi.org/10.20892/j.issn.2095-3941.2023.0002.
9 Li Y, et al. T Cell Receptor-Directed Bispecific T Cell Engager Targeting MHC-Linked NY-ESO-1 for Tumor Immunotherapy. Biomedicines 12(4) 2024: 776; https://doi.org/10.3390/biomedicines12040776.
10 Goebeler M-E, Stuhler G, Bargou R. Bispecific and Multispecific Antibodies in Oncology: Opportunities and Challenges. Nat. Rev. Clin. Oncol. 21(7) 2024: 539–560; https://doi.org/10.1038/s41571-024-00905-y.
11 Bratt J, et al. Therapeutic IgG-Like Bispecific Antibodies: Modular Versatility and Manufacturing Challenges, Part 1. BioProcess Int. 15(11) 2017: 36–42; https://www.bioprocessintl.com/manufacturing/therapeutic-igg-like-bispecific-antibodies-modular-versatility-and-manufacturing-challenges-part-1.
12 Morrison C. Nanobody Approval Gives Domain Antibodies a Boost. Nat. Rev. Drug Discov. 18(7) 2019: 485–487; https://doi.org/10.1038/d41573-019-00104-w.
13 Kitazawa T, Shima M. Emicizumab, a Humanized Bispecific Antibody to Coagulation Factors IXa and X with a Factor VIIIa–Cofactor Activity. Int. J. Hematol. 111(1) 2020: 20–30; https://doi.org/10.1007/s12185-018-2545-9.
14 Ding Y, Kumar H, Marino M. Antibody Purification Process Development and Manufacturing. BioPharm Int. 32(12) 2019: 24–29; https://www.biopharminternational.com/view/antibody-purification-process-development-and-manufacturing.
15 Bratt J, et al. Therapeutic IgG-Like Bispecific Antibodies: Modular Versatility and Manufacturing Challenges, Part 2. BioProcess Int. 16(1–2) 2018: 40–49; https://www.bioprocessintl.com/cell-line-development/therapeutic-igg-like-bispecific-antibodies-modular-versatility-and-manufacturing-challenges-part-2.
16 Sun Y, et al. Bispecific Antibodies in Cancer Therapy: Target Selection and Regulatory Requirements. Acta. Pharm. Sin. B 13(9) 2023: 3583–3597; https://doi.org/10.1016/j.apsb.2023.05.023.
17 Li Y, et al. A Roadmap for IgG-Like Bispecific Antibody Purification. Approaches to the Purification, Analysis, and Characterization of Antibody-Based Therapeutics. Matte A, Ed. Elsevier: Amsterdam, Netherlands, 2020; 167–179.
18 Wang LL, et al. Silencing Fc Domains in T Cell-Engaging Bispecific Antibodies Improves T-Cell Trafficking and Antitumor Potency. Cancer Immunol. Res. 7(12) 2019: 2013–2024; https://doi.org/10.1158/2326-6066.CIR-19-0121.
19 Portell CA, Wenzell CM, Advani AS. Clinical and Pharmacologic Aspects of Blinatumomab in the Treatment of B-Cell Acute Lymphoblastic Leukemia. Clin. Pharmacol. 5(S1) 2013: 5–11; https://doi.org/10.2147/cpaa.s42689.
20 WHO/MAB/DRAFT. WHO Guideline for the Safe Production and Quality Control of Monoclonal Antibodies for Use in Humans. World Health Organization: Geneva, Switzerland, 12 October 2021; https://cdn.who.int/media/docs/default-source/biologicals/mabs-manufacture-guideline-draft-for-1st-public-comment.pdf.
21 Dahodwala H, Lee KH. The Fickle CHO: A Review of the Causes, Implications, and Potential Alleviation of the CHO Cell Line Instability Problem. Curr. Opin. Biotechnol. 60, 2019: 128–137; https://doi.org/10.1016/j.copbio.2019.01.011.
22 Bork K, Horstkorte R, Weidemann W. Increasing the Sialylation of Therapeutic Glycoproteins: The Potential of the Sialic Acid Biosynthetic Pathway. J. Pharma Sci. 98(10) 2009: 3499–3508; https://doi.org/10.1002/jps.21684.
23 Mora A, Ezzyat Y. Anticipating Cell-Line Challenges To Drive CMC Readiness. BioProcess Int. 18(6) 2020: 52–58; https://www.bioprocessintl.com/cell-line-development/anticipating-cell-line-challenges-to-drive-cmc-readiness.
24 CMC Biotech Working Group. A-Mab: A Case Study in Bioprocess Development Study Guide, Version 2.1. CASSS: Sacramento, CA, 28 October 2009; https://www.casss.org/docs/default-source/biotech-working-group/a-mab_case_study_studyguide_v2-1.pdf?sfvrsn=f844b73_11.
25 Bas M, et al. Fc Sialylation Prolongs Serum Half-Life of Therapeutic Antibodies. J. Immunol. 202(5) 2019: 1582–1594; https://doi.org/10.4049/jimmunol.1800896.
26 Wong DCF, et al. Impact of Dynamic Online Fed-Batch Strategies on Metabolism, Productivity, and N-Glycosylation Quality in CHO Cell Cultures. Biotechnol. Bioeng. 89(2) 2005: 164–177; https://doi.org/10.1002/bit.20317.
27 Ehret J, et al. Impact of Cell Culture Media Additives on IgG Glycosylation Produced in Chinese Hamster Ovary Cells. Biotechnol. Bioeng. 116(4) 2019: 816–830; https://doi.org/10.1002/bit.26904.
28 Lewis AM, et al. Understanding and Controlling Sialylation in a CHO Fc-Fusion Process. PLOS One 11(6) 2016: e0157111; https://doi.org/10.1371/journal.pone.0157111.
29 EMA/CHMP/BWP/532517/2008. Guideline on Development, Production, Characterisation, and Specification for Monoclonal Antibodies and Related Products. European Medicines Agency: Amsterdam, Netherlands, 21 July 2016; https://www.ema.europa.eu/en/documents/scientific-guideline/guideline-development-production-characterisation-and-specification-monoclonal-antibodies-and-related-products-revision-1_en.pdf.
30 Chen SW, Zhang W. Current Trends and Challenges in the Downstream Purification of Bispecific Antibodies. Antib. Ther. 4(2) 2021: 73–88; https://doi.org/10.1093/abt/tbab007.
31 Gao B, Tsan M-F. Endotoxin Contamination in Recombinant Human Heat Shock Protein 70 (Hsp70) Preparation Is Responsible for the Induction of Tumor Necrosis Factor α Release by Murine Macrophages. J. Biol. Chem. 278(1) 2003: 174–179; https://doi.org/10.1074/jbc.M208742200.
32 ICH Q5C. Stability Testing of Biotechnological/Biological Products: Scientific Guideline. International Council for Harmonisation of Technical Requirements for Pharmaceuticals for Human Use: Geneva, Switzerland, 30 November 1995; https://database.ich.org/sites/default/files/Q5C%20Guideline.pdf.
33 21 CFR Part 600. Biological Products: General. US Food and Drug Administration: Rockville, MD, 2024; https://www.ecfr.gov/current/title-21/part-600.
34 ICH Q5A(R2). Guideline on Viral Safety Evaluation of Biotechnology Products Derived from Cell Lines of Human or Animal Origin. International Council for Harmonisation of Technical Requirements for Pharmaceuticals for Human Use: Geneva, Switzerland, 1 November 2023; https://database.ich.org/sites/default/files/ICH_Q5A%28R2%29_Guideline_2023_1101.pdf.
35 ICH E6(R2). Integrated Addendum to ICH E6(R1): Guideline for Good Clinical Practice. International Council for Harmonisation of Technical Requirements for Pharmaceuticals for Human Use: Geneva, Switzerland, 2016; https://database.ich.org/sites/default/files/E6_R2_Addendum.pdf.
36 CBER/CDER. Points To Consider in the Manufacture and Testing of Monoclonal Antibody Products for Human Use. US Food and Drug Administration: Rockville, MD, 20 April 2020; https://www.fda.gov/regulatory-information/search-fda-guidance-documents/points-consider-manufacture-and-testing-monoclonal-antibody-products-human-use.
Further Reading
Kim KS, et al. Clinical Pharmacology Strategies for Bispecific Antibody Development: Learnings from FDA-Approved Bispecific Antibodies in Oncology. Clin. Pharmacol. Ther. 116(2) 2024: 315-327; https://doi.org/10.1002/cpt.3308.
Corresponding author Yanhuai (Richard) Ding is senior director and head of CMC for biological DS process development and manufacturing; Bruce Andrien is senior director of analytical development; Mohosin Sarkar is director of biotherapeutics development; and Mark Aimone is senior vice president of CMC and manufacturing operations — all at EvolveImmune Therapeutics, Inc., 23 Business Park Drive, Branford, CT 06405; 1-413-687-1061; [email protected]. All trade names mentioned herein are registered with their respective owners.