In past decades, the focus in bioprocess engineering was on traditional stainless steel project management, which is highly dependent on established project schedules. Many milestones, tasks, and deliverables during basic and detailed design and execution were implemented in the same tried and tested way by engineering, suppliers, and biopharmaceutical companies. Making decisions was complicated by alignment with long lead items. With the introduction of single-use (SU) technologies, the transition in execution and optimization of project management only just has been fully understood. When SU technologies were first introduced, many experienced and established engineering companies tried to reuse their proven workflows for them. Now they recognize that the technical innovations also require a rethinking of project execution.
Today, there is great demand for adapting traditional bioprocess project management activities. Customers now specifically need flexible facility layouts and platform processing including a range of media/buffer preparation areas for extensive SU technology integration concepts (SUTICs). Also, the concept design including buffer distribution models now is influencing the complexity of today’s SU designs and requires an extensive understanding of possible technical solutions. Functionally closed-operation and sterile process transfer between unit operations and different cleanroom classes now are challenging process engineers with commitments and specifications they have to make at an early phase. All this is moving engineering work packages and procurement processes to an earlier project phase, leading the industry to change its traditional project execution models.
Facility Design Driven By “Process First”
From concept to realization, a single- or multiuse bioprocess facility always should be designed and built around process specifications, not vice versa. Since the emergence of SU technology, significant changes have occurred in facility design and engineering. Those most commonly seen include lowered capital costs, reduced facility footprints, elimination of sterilization-in-place (SIP) and clean-in-place (CIP) utilities, shortened build times, and increased footprint flexibility. What is less frequently acknowledged is the shift in engineering responsibilities and scenarios.
When it comes to SU facility design, typical engineering responsibilities are split between engineering contractors and SU equipment suppliers, both parties working closely together to ensure that all process needs are met. Engineering contractors typically take care of infrastructure, building, and good manufacturing practice (GMP) facility design, covering all business-related tasks and cleanroom arrangements; SU suppliers deal with process requirements and equipment integration. The shift from multiuse to SU systems allows more flexibility in a facility by making all efforts primarily process driven. The split of core competencies has brought benefits to users because SU experts can provide the high level of technological expertise required for both clinical and commercial manufacturing.
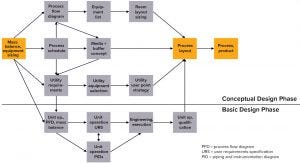
Figure 1: Mass balancing report (MBR) as the basis of engineering tasks at Sartorius Stedim Biotech
The basis of Sartorius Stedim Biotech (SSB) engineering tasks is the mass balancing report (MBR) tool (Figure 1), which provides all process relevant data. The basic factor is the throughput of drug per batch, which drives process titer because the amount of product upstream dictates the size of downstream equipment. Different titers for different processes also can lead to different requirements. Results generated by the MBR tool include all process-relevant data, such as process volumes for intermediates, antibody concentration at each unit operation, media and buffer demand based on column and filter sizes, and processing time slots for each individual step (which defines the process schedule). With this starting tool, facility design already is considered.
For example, the classical approach to buffer preparation and distribution was based on huge “tank farms” running vertically from the floor down into the downstream processing (DSP) rooms. Such installations were highly complicated and inflexible. SU allows for more of a one-floor concept in which mobile and stationary totes or Sartorius Palletank vessels hosting SU bags are used for buffer hold. In the best setups, those vessels are placed adjacent to process units, with tubing guided along the wall through systems such as Vortex Iris valves to minimize processing space and prevent movement within these critical areas. It is important to note that size and placement of buffer tanks must be considered from early design phases to ensure that a process runs smoothly.
Design According to Flow
Another key aspect in the early facility and process design phases is optimized flow of materials: material, waste, and personnel. Recently, “ballroom” or “dancefloor” concepts have emerged. Compared to the traditional approach, they have no fixed equipment, minimal segregation, and large open areas where mobile skids allow for rapid reconfiguration and construction, with all systems functionally closed and isolated from the environment. “Dancefloor” concepts differ in that they include small, compact, divided areas where clients want segregation to prevent movement of large volumes. This concept is becoming especially relevant as the market moves toward continuous processing, requiring large amounts of liquid. In ballrooms and dancefloor facilities, the flow of materials must be tracked carefully and coordinated to prevent processing errors — unlike the classical approach, in which units must be placed statically to support the correct flow of fluids, waste, and personnel to prevent cross contamination. In addition to the movement of material and personnel, correct disposal of solid waste (especially larger SU units such as bags and long tubing) must be considered. Material is an important consideration in waste disposal. For example, flexible tubing can contain just one type of polymer, whereas other products such as 3-D containers can include multiple types. SU systems can generate large amounts of waste in just one unit operation.
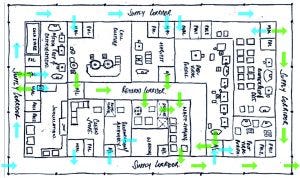
Figure 2: Room layout study including personnel flow
The main objectives in designing an SU facility are to minimize system complexity, support flexibility, and optimize the flow of materials, personnel, and waste. Figure 2 shows a room layout study, including personnel flow. It covers all unit operations in upstream and downstream lines. The layout is derived from a process flow diagram (PFD) that clearly defines all interconnections, and provides all major equipment sizing.It also forms a basis for the definition of consumables, which are key for SU processes, as well as a starting point for initial cost estimates for capital expenditures (CAPEX) and operational expenditures (OPEX).
Facility design is a complex, multistep process. Success requires knowledge of materials, equipment, consumables, and processes, and how they all work together. A strong collaboration and exchange with operators ensures a final acceptance. Parallel to process and facility design, it also is important to consider the need for process control based on advanced automation and data handling to maximize efficiency and minimize risk in biomanufacturing.
Automation and Data Analysis
Following recent trends in digitalization, usability, scalability, and system access, the industry is changing its approach to process automation. A focus on modular solutions with decentralized intelligence and digitalization is essential to enabling the required flexibility in SU processing. A major trend is integration of intelligent package units with process skids (e.g., for bioreactors, chromatography, or filtration) into process control systems such as Siemens PCS 7 into the overall automation landscape of a biopharmaceutical facility. This involves fulfilling batch-process control standards and GMP requirements for reporting, data management, user handling, auditing, and visualization. Full electronic batch records, review-by-exception methodology, and multivariate data analytics (MVDA) for real-time process monitoring, control, reporting, and release are being implemented to smart SU biomanufacturing facilities.
Bioprocess automation systems also can be aligned with the facility concept for building management systems (BMS) and the environmental monitoring system (EMS), for which data handling requires full qualification and validation. In selecting an automation strategy, it is important to address process needs because automation won’t help (and even could be detrimental) if core processes aren’t aligned.
Another emerging issue in SU-bioprocessing and automation is the use of real-time data analytics and MVDA process monitoring. In bioprocessing, MVDA is used to handle large, complex datasets generated in both development and manufacturing by process analytical technology (PAT). In research environments, MVDA can be used to support process development and optimization as well as classification of critical process parameters (CPP) operation windows and delivering critical quality attributes (CQAs). This provides quality analysis on decisions made and their associated risks and prevents deviations before they occur.
In commercial manufacturing, MVDA and online analytics deliver real-process monitoring and prediction based on models built with historical data. This not only enables corrective action and process intervention, but also provides performance insight and product quality assurance even before a batch is finished. Every second, thousands of data points are created and scattered across multiple systems, in a wide range of formats. Operators should know how a current bioprocess is running compared to optimal process parameters. MVDA models and real-time data analytics provide the necessary tools. In essence, PAT enables developers to build quality into a product and helps operators track how a current process compares to an optimized process (“golden batch” analysis).
SU Operation Challenges
Operational procedures for common process steps often differ significantly among end users, processes, and biomanufacturers. Media and buffer preparation, for example, follows well-known and accepted sequences that in principal can be covered by basic SU setups. The availability of a standard SU solutions for buffer or media preparation sounds straightforward enough. Nevertheless, available bag designs, tubing sets, and filter transfer sets differ among suppliers and end users both. Currently, >70% of stock-keeping units (SKUs) are custom made specific to just one customer — leading to high variability in raw materials and a complex supply chain with great potential for improvement in cost, logistics, and quality.
Essentially, “standardization” is not at issue; the challenge is that everyone wants their design to be the standard. The Standardized Disposables Design (SDD) initiative took an initial step toward designing simple standard SU solutions. Its mission is to facilitate standardization of designs among different biopharmaceutical companies to simplify the approach to selection and implementation. As an ultimate goal, “each predesigned SU assembly would be prequalified to a defined ‘design space,’ which outlines its process capabilities” (e.g., operating limits, extractables profile, and so on).
Implementation of SU projects with standardized consumable options would allow for a predictable course of the consumable part of the project because the functionality and setup will have been proven. Different configurations would be prequalified, and interaction between SU assemblies, process hardware, and software are considered fully. For users, having standardized SU solutions would improve assurance of supply, make manufacturing leaner, eliminate design time and qualification costs, and eventually speed products to market.
This occurs especially when the risk of affecting timelines grows as the complexity of project execution increases. In most cases, SU bioprocess equipment looks simpler than traditional stainless-steel technologies at first, especially as by eliminating CIP/SIP. This goes hand-in-hand with the expectation that SU significantly reduces overall project timelines. All this is possible only with a move to change traditional execution models. It is important to understand that different project phases cannot take place sequentially; they have to run in parallel. Previously, long-lead items such as frames and major components were required to begin the assembly process. They were followed by system piping and then electrical and pneumatic installation. That allowed project teams to focus initially on long-lead items and the basic design phase (Figure 1) before discussing in the detail design specific solutions for piping routing and arrangement of components.
The Need for Standardization
Although the benefits and drivers of SU technologies are well known in bioprocessing, it is important to understand their limitations and evaluate alternatives in early project phases (hybrid processes). It might seem obvious, but a process running in stainless steel skids cannot be copied simply step by step into a SU process: Limits in scale, availability of sensor technologies, and achievable flow rates must be considered early on.
With implementation of SU technologies, additional quality-related topics such as extractables and leachables, closure integrity, or particulate matter come into play. Currently, only a few standardized protocols are available for evaluation and documentation of quality attributes for SU processes. Standards agencies and end-user forums including the BioPhorum Operations Group (BPOG) are working on establishing test methods for SU components. Once available, those protocols will be valuable not only to end users and vendors, but also to regulatory agencies.
After technical aspects, dependency on suppliers’ delivery performance and quality play a major role (along with cost). The industry is aware of the advantages and disadvantages of supplier dependency and is focused on operational supply chain management, including second sourcing and supplier relationships. This is highly important with respect to economic pressures on warehouse management, demand planning, and delivery reliability, which has led to a need for standardization of SU operations. Many initiatives have begun over the years, but the most promising is the Standardized Disposable Designs (SDD) campaign that introduced new designs based on off-the-shelf components. They are helpful for supply chain management (SCM), enabling secondary sourcing and supply assurance with multiple suppliers. Nevertheless, the root of the problem remains: uncontrolled variance in single-use assemblies. That creates highly complex SCM, logistics, purchasing, operations, and operator training because of a significantly high number of different consumable sets for processing.
Thus, it is critically important to develop variant management from the start, investigating different variants while trying to keep them at a minimum and establish a standardized process toolbox with functional groups. A good example would be a pumping line with pressure measurement, mostly used for transfer but also for filtration purposes. Such a line could have a standardized interface with a predefined length. Whenever a longer distance for transfer is required, a toolbox of extension lines could be made available to span the distance. This concept would increase efficiency while reducing cost of goods (CoG) and would improve warehouse management, operations, and SCM, while reducing process risks for operators who would not have to become familiar with many different SU configurations. First evaluations have shown a potential for reducing the process toolbox to only 30% of today’s different consumable setups.
The Future: “Batch in a Box”
With increasing implementation of different SU systems and components, identification and correct installation of SU subassemblies within a process has become a major issue. With each additional SU assembly, the risk of connecting the wrong sets to each other increasing, potentially compromising the overall process. When an error is observed in time, a setup can be fixed if port disconnection and reconnection is possible (e.g., by triclamp connections). Aseptic connections, however, are typically irreversible. Consequently, the wrong connection makes a setup (or parts of it) useless. This problem becomes even more costly when expensive components such as chromatography columns, tangential-flow filtration (TFF) cassettes, virus filters, single-use sensors, or pump heads are incorporated into the assemblies.
Currently, few vendors offer solutions to guide users into making the correct connections for their intended setups. Available identification methods vary from color coding or radio frequency identification (RFID) tags on tubing ports to “blueprints” showing the routes of tubing, sensors, and connectors. A widely accepted methodology has not yet been established. So a quick, secure method of identifying which consumable connections fit with each other remains a challenge that the industry must solve.
SCM also will become more important to optimize shipping, storage, and capital considerations. Batch picking of consumables could be one improvement. Today many are delivered in minimum quantities, and operators or internal logistics staff must unpack, pick, and transport batch-related consumables to operation areas. That requires time and expense, and it increases the risk of damage from additional handling. A “batch-in-a-box” concept would guarantee batch-wise packed consumables based on a functional toolbox. They could come with emergency consumable sets with the most critical components for batch recovery. That would ease SCM processes significantly and improve warehouse management and demand planning while allowing suppliers to manage their production more effectively.
All these approaches would require updated project execution models and new training strategies. Companies need to be able to do the right thing at the right time and use the benefits of fast-track project execution — one of the main reasons for implementing SU processing.
A New Paradigm
SU technologies have changed how biomanufacturing plants are designed and constructed. The requirement for much more flexible layouts with ballroom and dancefloor concepts has led to facilities being constructed around process specifications. Mass balance, material flow, automation integration, and data analytics all need to be considered in different ways. The amount, types, and scale of SU technologies have changed and increased significantly, adding an extra burden on facility design and operations.
One solution to many current SU technology issues is standardization, and the SDD initiative is beginning to have an impact. Improved management of variance is another approach for designing and operating SU technologies more efficiently. However, a future strategy would be to deliver a “batch in a box,” with all SU components for a given batch process packed and delivered together. That concept would require biopharmaceutical companies to change their thinking about bioprocess project execution models, but it also would allow them to achieve the maximum efficiency and operational benefits of SU technologies.
Corresponding author Jan Schäfer is manager of process consulting and engineering (49-5665-407-1226, jan.schaefer@ sartorius-stedim.com); Michael Koch is head of sales for applied bioprocessing; Dr. Jens Landmann is project manager; and at the time of this writing, Stefanie Beck was a consumable expert — all in Integrated Solutions at Sartorius Stedim Biotech.