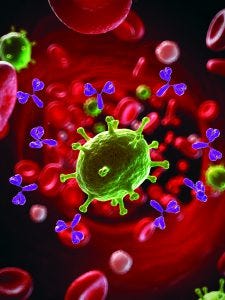
The function of a MAb depends on its binding to a cell surface. (STOCK.ADOBE.COM)
The modern age of biologics began 35 years ago with the approval of Lilly’s Humulin product — a biosynthetic form of human insulin derived from recombinant DNA and microbial cell culture (1). Today, about a quarter (27%) of the drugs approved yearly by the US Food and Drug Administration (FDA) and European Medicines Agency (EMA) are biopharmaceuticals: primarily monoclonal antibodies (MAbs), but also vaccines, blood products, and (recently), advanced therapies based on genes and cells. A decade ago, the average annual approvals of biopharmaceuticals made up only 13% of overall products approved (2).
One industry-representative organization, the Pharmaceutical Researchers and Manufacturers of America (PhRMA), has said that more than 900 biologics targeting cancer, infectious diseases, autoimmune conditions, and heart problems are in development (3). More than 50 investigational MAb therapeutics currently are undergoing evaluation in late-stage clinical studies. Those are expected to drive a trend toward first-marketing approvals of at least six to nine MAbs per year in the near term, according to a recent paper by The Antibody Society (4). And Transparency Market Research, a New York analysis firm that follows the drug industry, predicts that the global biologics market will exhibit a compound annual growth rate (CAGR) of ~11% between now and 2020, more than doubling the revenues — from US$209 billion to $480 billion — for biomanufacturers (5).
Reflecting those dramatic changes comes a rise in the number of biosimilars in development, products that regulatory agencies generally define as being similar to a biological reference and having demonstrated no clinically meaningful differences from it. The EMA approved the first biosimilar in 2006 — a human growth hormone — and the first biosimilar MAbs in 2013. Now 23 biosimilars are sold in Europe. The United States was slower to develop a regulatory pathway for biosimilar approval but finally approved its first two years ago: a drug intended to help prevent infections in chemotherapy patients. Presently, four biosimilars have been approved for the US market.
Biosimilars are poised to have an even bigger impact on both European and US markets, with MAbs representing the most attractive targets for developers of these follow-on products. As patents and data-protection measures expire, particularly for therapeutic proteins that rank in the top ten of revenue-producing pharmaceuticals, biosimilar companies will want to make copies that are more affordable and can improve global access to otherwise expensive blockbuster drugs. Over a dozen US and EU companies have 30–40 biosimilar molecules in late-stage clinical trials. An estimated 100–200 emerging-market companies are engaged in trials at different stages with biosimilar products, according to a 2015 report by the Tufts Center for the Study of Drug Development (6).
But developing biologics and particularly biosimilars is not easy, with a much more complicated regulatory process than that for small-molecule drugs. The size of biotherapeutic molecules and the complexity of materials and methods used to make them far outpace those of generic drugs. Proteins are usually 100–1,000× larger than small-molecule drugs and can’t be fully characterized physicochemically with current analytical methods, which are often insufficiently sensitive. Their modes of action also can confound in vitro biological characterization studies (7).
MAb molecules have different functional domains, such as the variable antigen-binding region and the constant domains that interacts with Fc receptors and bind with complement proteins. Such complexity can make comparability studies between reference products and biosimilar candidates a difficult hurdle for developers to negotiate because it is impossible for two different manufacturers to produce two identical products — even using identical host expression systems, processes, and equivalent technologies. Even minor variations in production protocols can affect the posttranslational modifications of an antibody therapeutic and thereby change its biological activity.
Assays for Measuring Function: To determine whether such therapeutic compounds perform effectively, bioassays play a critical role in drug discovery and development by helping developers measure the activity of biologic candidates. For biosimilars, regulators require biocomparability testing by comparing candidate compounds with different lots of innovator molecules sourced from different markets. A well-developed bioassay can enable accelerated stress-condition testing and short-term dilution stability studies (e.g., using IV bags). Even the confirmation of successful process scale-up can be supported with in vitro bioactivity assays, although that is not a regulatory requirement.
Drug companies need suitable bioassays in the earliest stages of product development — often more than one assay for MAbs because they can have multiple mechanisms. These assays need to be reliable, reproducible, and precise.
Noting the changing landscape in biologics development and the growing pipeline of biosimilar candidates, the World Health Organization (WHO) added a proposed product-specific document last year to its existing guidelines (8). The 28-page draft offers special considerations for MAbs, such as using surrogate ligand-binding assays (rather than classical bioassays) to estimate their potency if it is known for certain that binding is the major mechanism of action (MoA). It can be difficult to identify or produce appropriately responsive target and efficient effector cells. Because assays measuring Fc function can be technically demanding the draft states that companies can use continuously growing cell lines as a replacement for cells from primary sources. Engineered cell lines are appropriate, and different data may be generated using different assay formats, cells, or cell combinations. The FDA has issued recent guidelines on assay development and validation for immunogenicity testing of therapeutic protein products in clinical studies (9).
Many therapeutic antibodies on the market — especially anticancer MAbs — follow classical pathways related to the innate human immune response. One example is the complement system, which enhances or complements the ability of antibodies and phagocytic cells to clear microbes and damaged cells, promote inflammation, and attack a pathogen’s plasma membrane. It includes a complex cascade of activating and regulatory principles involved in early recognition of things that the body recognizes as foreign.
Our immune systems use a number of well-documented MoAs to single out and kill specific pathogens and cancer or infected cells: antibody-dependent cellular cytotoxicity (ADCC), complement-dependent cytotoxicity (CDC), and programmed cell death (apoptosis). Antibody-dependent cellular phagocytosis (ADCP) is a less studied and more circuitous MoA. Assays that measure these classical pathways are the favored method of determining the potency, safety, and effectiveness of therapeutic antibodies. They also are time-consuming, tedious, and sometimes highly variable in results when comparing biosimilars with reference materials. Thus, we are seeing the rising use of surrogate assays wherever primary-cell–based, MoA-reflecting assays are inappropriate.
The Classical Testing Route
Functional assays measure biological activity throughout the lifecycle of all MAb products, providing a means for sponsor companies to ensure that these drug candidates are safe, effective, and high enough in quality to progress. Here, drugs are analyzed systematically and compared with well-defined reference materials in the presence of appropriate positive and negative controls. Regulatory authorities require use of functional assays that reflect a compound’s MoA, such as how it binds to an intended molecular target to induce a certain in vivo immunological pathway to produce a therapeutic effect. Assays that reflect the MoA are relevant for final clone selection and required for lot release, as well as in stability testing, to determine the bioactivity of each individual sample for biocomparability.
ADCC-mediating antibodies act as a bridge between an infected cell or tumor cell and immune cells. This MoA requires that the tips of an antibody bind to a target cell while the other end of the molecule binds to proteins on the surface of natural killer (NK) cells. Those then kill target cells by releasing preformed toxic proteins through a mechanism that secretes and then discharges the proteins outside target cells. ADCC-based assays are well-regarded because they best reflect that in vivo situation, but they are tedious, time-consuming, and expensive. They also depend on the quality of NK donor cells. Fortunately, acceptance is rising for continuously growing artificial effector cells and engineered effector cells with reporter readouts, even though they cannot fully replace primary assays for MoA confirmation.
CDC is induced when a protein complex known as C1q binds an antibody. That triggers formation of a membrane attack complex that punches holes in the surface of target cells. Apoptosis occurs when a family of enzymes inside a target cell sets off a cascade effect after the MAb binds to its target on the cell surface (10). In ADCP, receptors on macrophages or other phagocytic cells bind to antibodies that are attached to target cells, including tumor cells. Macrophages are crucial to the effectiveness of many antibodies in tumor therapy because they perform the process of ADCP (11).
Different functional bioassays analyze those different MoAs. ADCC assays use target cells that express antigens specific to the pathogen that an antibody is targeting, effector cells such as NK cells, and an antibody source (e.g., serum, plasma, or purified therapeutic MAbs). ADCC assays measure the death of target cells effected by NK cells in the presence of antibodies, usually by measuring the amount of a dye or other compound that cells release when they die (12). CDC assays use flow cytometry or luminescence methods, with complement proteins from a commercial source (e.g., baby rabbits), to estimate tumor damage and measure the functionality of pathway induction by reading MAb dose-dependent cell death caused by formation of a membrane-attack complex.
ADCP is much more difficult to measure using primary macrophage cells. Those macrophages must be derived from primary monocytes isolated from blood donors. Unlike NK effector cells, they need to be differentiated in culture to become macrophages for nearly a week before use. Effector and target cells then must be made distinguishable through use of a pH-sensitive dye to label target cells and a marker that is specific for the macrophage effector cells. Flow cytometry then generates the response curves based on the results (13). This is an extremely complex and time-consuming process. Many laboratories simply don’t have the trained staff or equipment to run such assays. The two-step process of measuring ADCP makes the use of flow cytometry challenging. The assay’s dynamic range also is limited, even for therapeutic antibodies known to be strong inducers of ADCP.
Another method of measuring ADCP is with a reporter-gene assay, which can be used to assess the activity of a particular promotor in a cell or organism. For this approach, primary macrophages are replaced with an engineered reporter cell line that stably expresses FcgRIIa and NFAT-RE/luc2 proteins. As with primary macrophages, the same signaling for ADCP is activated when the FcgRIIa is bound by an antibody that’s bound to a target cell — meaning that the reporter assay reflects the in vivo molecular pathway for phagocytosis by macrophages mediated by Fc-receptor proteins. This surrogate bioassay solves some of the problems found with primary assays: It eliminates the need to source, purify, and perform multiday cytokine differentiation of macrophage effector cells as well as the variability of different primary cell donors.
Bioassays for Checkpoint Inhibitors
Major conceptual and technical advances over the past 30 years have opened the door to powerful drugs that harness our own immune systems to fight cancer. One promising strategy involves blocking immune checkpoint molecules such as CTLA4 or PD-1. By contrast with the earliest anticancer therapeutic MAbs, these newer MAbs interfere with the adaptive immune system and can activate both innate and adaptive immune responses.
Checkpoint molecules are proteins on T cells that act as a kind of cautionary brake that shuts down the cells and prevents them from “going rogue.” Tumor cells can hijack the checkpoint pathways to prevent attacks from the immune system, and many scientists believe — and in some cases have shown — that disabling immune checkpoints helps reengage the body’s immune system to fight against cancer. Examples of checkpoint-inhibiting therapeutic MAbs include pembrolizumab (Keytruda) and nivolumab (Optivo), which inhibit programmed cell death, and ipilimumab (Yervoy), which inhibits CTLA4 (14–16).
Checkpoint inhibitors are among the hottest oncology drugs in the pipeline, with more than 100 experimental CTLA4 inhibitors in development alone and dozens of other proteins considered to be potential targets. The next generation of cancer immunotherapies is aimed at combining these drugs with bispecific MAbs or cytostatic agents such as antibody–drug conjugates.
Because of the complexity of adaptive immune responses, primary assays can be very challenging for this drug class. The coactivating signal of antigen-presenting cells must be mimicked to achieve activation, and primary T cells are required to serve an effector function. Readouts of such assays can include cytokine release on activation, T-cell proliferation, target cell death, or flow-cytometric analyses of the expression of certain cell-surface markers that indicate differentiation toward an activated subtype. Those types of assays are highly relevant yet problematic because of the multiple cell types used in each assay, long assay times in some cases, and variability in sources of primary cells. Thus, such assays are insuitable for routine testing in a good manufacturing practice (GMP) environment.
Immune checkpoint reporter-based bioassays can provide a simple, consistent, reliable, and pathway-specific cell-based option to measure antibody function throughout drug research and development. Examples include blockade assays and surrogate reporter assays.
Blockade bioassays are used for PD-1/PD-L1 checkpoint inhibitors. One method uses PD-1 effector cells and target cells expressing both human PD-L1 and an engineered cell-surface protein designed to activate cognate T-cell receptors (TCRs) independent of antigens. When the two cell types are co-cultured, the interaction of PD-1 and PD-L1 inhibits TCR signaling and luminescence. Addition of an antibody against either PD-1 or PD-L1 to block the PD-1/PD-L1 interaction releases an inhibitory signal, resulting in TCR activation and luminescence.
A CTLA4 reporter assay my company uses involves a modified human T-lymphocyte cell line as CTLA4-expressing effector cells along with antigen-presenting cells that are needed for costimulating factors. The effector cells express luciferase depending on the CTLA4 down-regulating pathway. If CTLA4 is blocked, luciferase is expressed steadily and can be measured using luminescence plate readers.
Surrogate reporter assays reflect the MoA of pathway-specific therapeutic antibodies in a consistent format that works for GMP bioactivity testing. Surrogate reporter assays also are stability-indicating and suitable for high-throughput biosimilarity assessment. Surrogate assays are accepted by most regulatory authorities.
With regard to induction of classical immunological pathways such as ADCC and ADCP, reporter assays also can be useful in assessing the function of checkpoint inhibitors. Therapeutic MAbs such as those that interfere with T-cell signaling have the same features as all other therapeutic MAbs: They might be capable of mediating one or more MoAs in the classical immunological pathway group through their Fc regions. For example, ipilimumab can mediate ADCC, which is shown clearly with a CTLA-4 expressing target cell line and a surrogate ADCC-reporter effector cell line.
Not to Be Taken Lightly
Surrogate assays are stability indicating and suitable for biosimilar assessment. In the context of immune-checkpoint MAbs developed as biosimilars, classical immunological pathways might be relevant, too. It’s important to manage our expectations no matter which type of bioassay is used to measure the functional responses of therapeutic antibodies. Based on models with primary cells, these tests are extremely challenging. All are highly relevant, but they also can be problematic because of multiple cell types used, long assay-performance times, and variability in the sources of their primary cells.
References
1 Human Insulin Receives FDA Approval. FDA Drug Bulletin 12(3) 1982: 18–19; www.ncbi.nlm.nih.gov/pubmed/6762312.
2 Serebrov M. Biologics Share of Medicine Chest Grows, and So Do the Pricing Concerns. BioWorld, www.bioworld.com/content/biologics-share-medicine-chest-grows-and-so-do-pricing-concerns.
3 Biologics Research Pushing Frontiers of Science with More Than 900 Medicines and Vaccines in Development. Medicines in Development: Biologics. Pharmaceutical Researchers and Manufacturers of America: Washington, DC, 2013. http://phrma-docs.phrma.org/sites/default/files/pdf/biologics2013.pdf.
4 Reichert JM. Antibodies to Watch in 2017. MAbs 9(2) 2017: 167–181; doi:10.1080/19420862.2016.1269580.
5 Global Biologics Market Revenue. Transparency Market Research: Pune, India, 5 October 2016.
6 Outlook 2015. Tufts Center for the Study of Drug Development: Boston, MA, 2015; http://csdd.tufts.edu/files/uploads/Outlook-2015.pdf.
7 Schellekens H. How Similar Do Biosimilars Need to Be? Nature Biotechnol. 22, 2004: 1357–1359; doi:10.1038/nbt1104-1357.
8 Draft: Guidelines on Evaluation of Monoclonal Antibodies As Similar Biotherapeutic Products. World Health Organization: Geneva, Switzerland, March 2016; www.who.int/biologicals/MAb_1st_draft_KG_IK_1_March_2016_clean.pdf.
9 Notice of Availability: Assay Development and Validation for Immunogenicity Testing of Therapeutic Protein Products. US Food and Drug Administration: Rockville, MD, 20 April 2016; www.gpo.gov/fdsys/pkg/FR-2016-04-25/pdf/2016-09449.pdf.
10 Herbrand U. Choosing the Right In Vitro Test for Your Monoclonal. Gen. Eng. Technol. News 31 December 2013.
11 Kipp W. Macrophages Are Critical Effectors of Antibody Therapies for Cancer. MAbs 7(2) 2015: 303–310; doi:10.1080/19420862.2015.1011450.
12 Von Bubnoff A. Antibodies: Beyond Neutralization. IAVI Report January–February 2010; www.iavireport.org/vol-14-no-1-jan-feb-2010/1005-antibodies-beyond-neutralization.
13 Herbrand U. Antibody-Dependent Cellular Phagocytosis: The Mechanism of Action That Gets No Respect. BioProcessing J. 15(1) 2016; www.bioprocessingjournal.com/index.php/article-downloads/703-j151-antibody-dependent-cellular-phagocytosis.
14 Reck M, et al. Pembrolizumab Versus Chemotherapy for PD-L1-Positive, Non-Small-Cell Lung Cancer. NEJM 375, 2016: 1823–1833.
15 Borghaei H, et al. Nivolumab Versus Docetaxel in Advanced Non-Squamous Non-Small-Cell Lung Cancer. NEJM 373, 2015: 1627–1639.
16 Hodi FS, et al. Improved Survival with Ipilimumab in Patients with Metastatic Melanoma. NEJM 363, 2010: 711–723.
Ulrike Herbrand, PhD, is a scientific supervisor for research and development (R&D) in biosafety and bioassay services at Charles River Laboratories’ Biologics Testing Solutions, Max-Planck-Straße 15A, 40699 Erkrath, Germany; 49-211-9255-332; [email protected]; www.criver.com. She presented on the topic of this article at the 2016 BioProcess International Conference in Boston.