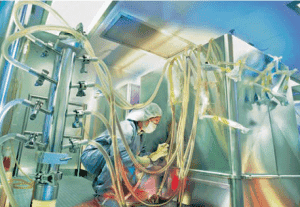
Factor VIII production at Bayer’s facility in Berkeley, CA (www.bayer.com)
With 20 years of experience in the biopharmaceutical industry — at Genentech, Applied Biosystems, Cell Genesys, Cellerant Therapeutics, and Bayer — Yuval Shimoni has written frequently for BioProcess International on a number of production topics. Those have ranged from process improvements and bioreactor scale-down validation, to raw materials management, to addressing variability and virus contamination events. For this featured report, we discussed hardware and instrumentation, quality by design (QbD) and related approaches, and other strategies that can take expediting upstream process development beyond just cell-line development.
Single-Use Technologies
Disposables enable continuous/perfusion culture, among other things. How do they contribute to a strategy of speed to the investigational new drug (IND) application? The two distinct production platforms most commonly used in the biopharmaceutical industry are fed-batch and continuous/perfusion culture. The choice of platform has been driven traditionally by considerations ranging from the nature of a given product (e.g., labile proteins are more protected from degradation by continuous harvesting) to the platforms and infrastructure available to a given sponsor company.
Most companies have more experience with fed-batch systems. But . . . ? Availability of single-use bioreactor (SUB) perfusion cell culture systems and accessories — including single-use, disposable cell-retention devices — is making an even better case for perfusion as the system of choice (1). This is especially true for younger companies — those that have yet to reach the investigational new drug (IND) application stage. On one hand, they are not tied to expensive (to purchase, run, and maintain) preexisting stainless steel systems. They also tend to be space limited.
Many companies that are not already invested in stainless steel platforms — and/or have limited space available for production of pre-IND material — are attracted by relatively small up-front costs and footprints afforded by single-use systems (compared with stainless steel). Multiuse platforms need to be cleaned and sterilized between campaign runs, which entails validation of all steps involved. Elimination of that validation reduces complexity and contributes to faster progress toward IND filing. This is of highest importance to sponsors in the pre-IND period, during which resources are limited.
Automation
I’ve seen some impressive shortening of cell-line development timelines through automated clone-picking and nanoscale technologies. I’ve also heard a lot of talk about microscale bioreactors (e.g., the ambr system from Sartorius Stedim Biotech) in early upstream process development. How are such technologies changing the game? Automation has played a game-changing role to conserve labor and time at all stages of biopharmaceutical development, including upstream screening and optimization. It also can increase development speed by linking clone selection to bioprocess optimization (e.g., control of physicochemical parameters and nutritional requirements for cell growth, productivity, and quality of expressed products). Moreover, automation facilitates improvements in product safety by reducing/eliminating exposure to animal sources (other than the host cells) through use of animal-free semisolid media for cloning — and by improving our ability to demonstrate and document clonality. Automation facilitates consistency while reducing ambiguity, cost, and labor in streamlined processes.
The labor-intensive days of clone screening and selection, involving serial dilutions and manual pipetting, have been transformed through introduction of high-throughput (HT) automated instruments. Likewise, traditional pilot-scale systems (e.g., benchtop bioreactors, shake flasks, and spin tubes) used for early process development, optimization, and initial product characterization have been replaced: Scale-down microbioreactors are much more representative of the production-scale platform than the earlier small-scale systems ever could be.
HT clone-selection instruments increase speed to identification of the most promising clones — and superior clones that come from a much larger pool for selection than otherwise would be possible. When coupled with image-capturing technologies, automation allows us to demonstrate single-cell clonality confidently. We can trace the origins of our ultimate choice for cell banking and use in production to a single transfected parental cell. This also increases consistency, which can significantly increase speed by reducing the need for repeat-experiments to meet product titer and quality goals.
Today’s microscale bioreactors are scaled-down proportionally in critical dimensions (e.g., aspect ratios and volume/surface-area ratio) to represent culture performance more faithfully at production and commercial scales. This is largely because automation enables on-line data collection for physicochemical variables such as temperature, pH, and dissolved oxygen (DO) and automatic adjustment of microbioreactor inputs (e.g., mixing, aeration, and cooling/heating). Commercially available microbioreactors are updating processes based on early semicustomized systems (2). Microbioreactors are replacing not only scaled-down batch modes, but also traditional small-scale perfusion-mimic systems that used media exchanges and centrifugation for cell settling in lieu of cell-retention devices. New methods help developers predict large-scale performance faithfully, including characteristics such as cell-specific perfusion rates, harvest rates, and links to downstream processing units.
High-Throughput Screening
Similarly, we’ve seen HT approaches for adapting cell lines to serum-free media. What can such screening technologies do for media optimization in process development? With semisolid media and better performance-representative scale-down cell culture platforms (microbioreactors), we have mitigated a key source of inconsistency: that resulting from shifts in relative ranking of clones when tested during screening and after scale-up. Under traditional methods, serum was added to selection media for promoting cell adhesion, which in turn facilitated single-cell isolation and further cloning. Clones that appeared to be the most promising (e.g., having the highest specific productivity) from such processes could not be counted on to maintain their respective ranking once adapted to serum-free conditions. Other disadvantages of using serum come from its being an animal-sourced raw material that introduces potential safety risks, being chemically undefined, and bringing variability from batch to batch. The ability to use the same serum-free media throughout clone selection, process scale-up, and development removes that source of inconsistency, significantly increases speed, and eliminates potential risks from exposure of cells to serum. The increased pace is achieved with a larger cohort of potential clones serving as a back-up reserve.
Qualified scale-down platforms are essential to rapid process development (toward an IND filing) because they allow for HT progression toward identifying optimal conditions (3). Media optimization is a good example in which reliable empirical performance can leverage design of experiments (DoE) methodology. A DoE approach leverages HT systems to shorten the time required for identification of optimal conditions (e.g., media composition) while providing an acceptable confidence level (2). We’ll have more confidence in our results the more representative our screening system is of production scale. HT systems are essential for testing many conditions by performing many experiments. (Typical cell culture media can have a large number of components each to be tested at different concentrations).
The desired outcome can be high growth, productivity, and/or meeting critical product quality attributes (PQAs). Meeting such goals quickly and reliably calls for two key requirements: a HT system (to run many cases in a relatively short time) and full scalability (to make sure that the identified optimum is scale independent). Scalability improvements such as those have been seen recently in fed-batch microbioreactors are sure to follow with scale-down perfusion systems: bioreactors, retention devices, and continuous controls.
Process Analytical Technology
Is on-the-floor/in-process (at-/on-line) testing vital for such advancements? What difference can it make in timelines? And is there room for improvement in such technologies? Process analytical technology (PAT) measures and analyzes critical process parameters (CPPs) to control critical quality attributes (CQAs). In-process analysis (e.g., through sensors placed in the process/in-line or connected to the process/on-line) circumvents additional processing called for with off-line and at-line analyses, which are characterized typically by (often manual) sampling followed by discontinuous sample preparation, measurement, and evaluation. In/on-line analysis generates data more rapidly, providing continuous and nearly instantaneous feedback to enable maintenance of CQAs within target ranges. Moreover, PAT eliminates the potential for changes in sample properties during the time from sample collection to results generation — thus increasing fidelity. This in turn allows us to make selective, optimized, and immediate adjustments/corrections to CPPs — before those changes would affect PQAs.
Prior knowledge and process understanding are critical to optimal programming of that feedback loop. Even if a process is new, implementing a baseline PAT can provide a major advantage toward producing consistent product quickly. That is especially important to controlling the greater variability inherent to processes that are still under development (for reasons ranging from supply of raw materials to changes in equipment). Thus, leveraging PAT and continuous production clearly speeds up milestone achievement: to the IND, the biologics license application (BLA), and beyond.
Today’s bioprocesses can collect enormous volumes of data. One area that needs significant improvement is process analysis software (e.g., for statistical multivariate data analysis) that can manage those volumes and make sense of the data. Other avenues for improvement include sensor technology adaptations for in-/on-line process analysis (e.g., near-infrared and Raman spectroscopy), and hardware interfaces (e.g., touchscreens for user friendliness). The former would facilitate determination of which product attributes are CQAs and which parameters are CPPs. The latter would simplify data collection, increase data fidelity, and improve control aspects. More knowledge gained through continuous data acquisition and analysis should use statistical software leveraging powerful approaches (e.g., DoE) to drive modern science- and risk-based approaches that enable fast continuous improvement.
Platforms and Prior Knowledge
Even as new methods and approaches are changing the industry, prior-knowledge assessments remain important. What kinds of upstream platform approaches help to speed things along? Platforms of choice and prior knowledge within organizations are linked intimately. The experience that subject-matter experts (SMEs) bring with them is the primary source of technical considerations for platform selection, notwithstanding other considerations such as preexisting infrastructure and space availability. A current shift we are seeing in the industry now is increased appreciation for the advantages brought by continuous systems: speeding development and reducing operational costs (pre-IND through production scale). Perfusion systems (such as those pioneered over three decades ago by Bayer in Berkeley, CA) were used commercially as early as the 1990s — but by only a handful of companies. The renewed interest in perfusion comes from gained knowledge and requirements for fast development.
Technical reasons include relatively low startup capital costs, smaller footprint demands, increased commercial availability and choice of equipment and technologies (e.g., retention devices and single-use equipment, parts and sensors), and a growing need for HT production. Cell and/or product-specific reasons to choose perfusion over fed-batch culture include the need for consistent availability of nutrients to cells with continuous removal of accumulating inhibitory byproducts (ammonia, lactate) and the ability to reduce product exposure to proteases. Meanwhile, earlier perceived disadvantages are dissipating: e.g., complexity of operations, risk of contamination, reliability and the cost of failure, and even availability of expertise.
Crossfunctional Teams
Should companies include downstream experts in upstream decision-making — and vice versa? What other functions can contribute to upstream process development efficiency? The practice of assigning independent teams to given responsibilities increases accountability and is important in commercial production, in which those teams are members of departments with defined roles and responsibilities. However, in settings such as investigations and process development, broad skills can improve chances greatly for success. “User Requirements” documents provide guidance to developers on expectations for a given process from a downstream receiving group. That remains at the level of “what,” however; for the “how,” a dialog is well worth the time because it provides an avenue for mutual understanding. For upstream process developers, it helps elucidate the difference between what is a “must” and what is flexible or “nice to have.” For downstream process folks, understanding and keeping abreast of ongoing upstream challenges can trigger new ideas for innovative solutions to accommodate those challenges.
Not considering and addressing the needs of downstream processing is a missed opportunity for upstream groups that can lead to unnecessary changes later on — in turn extending overall timelines and cost. For a better chance at “getting it right the first time,” upstream process developers can benefit greatly from including downstream process experts. A crossfunctional team should expand to include additional stakeholders as relevant and at a frequency that makes sense (allowing for timely addressing of feedback). Quality assurance (QA) and regulatory affairs (RA) are important stakeholders in a regulated environment because they could raise sets of requirements that otherwise might not have been considered — related to compliance and/or health-authority expectations, for example. A good practice for upstream developers might be to check in with all stakeholders, even those who might not seem obvious, and solicit their feedback after explaining the project. You might find it surprising how many times new considerations (that could lead to process modifications) arise by including stakeholders who often get brought in too late such as
procurement (materials availability)
supply chain (security of supply)
engineering (facility considerations)
quality control (QC, status of in-house/contracted test methods)
management (business considerations).
Quality By Design
Many companies wait to implement QbD later in product development — e.g., during clinical testing, when good manufacturing practices (GMPs) must apply. But can it help to include risk assessment and identifying quality attributes in early development — or does that inevitably slow things down? There are several aspects to consider in determining whether applying QbD makes sense. The key to speeding up IND submission without compromising the chemistry, manufacturing, and controls (CMC) package is an ability to justify minimum requirements at each development phase.
Consider the scope and quality of available data and process controls: Were data generated under GMP conditions using validated processes and qualified equipment? That will affect the regulators’ confidence in its being representative of the locked-in process as well as your ability to leverage a risk-based approach later on.
QbD requirements also affect cost/benefit decisions. The additional work and cost that QbD entails should pay off because higher levels of product and process understanding will increase success and robustness during development and manufacturing. This is important because a more robust process would have fewer incidences of (unintended) deviations and less administrative compliance follow‑up such as issuing deviation reports (DRs), conducting investigations, and implementing corrective and preventive actions (CAPAs). Continuously augmenting the design space (narrowing or widening it) as experience is gained will facilitate (and could reduce) postapproval submissions as well.
We’re now in the second decade since ICH Q8(R2) became effective, with its intention “to design a quality product and its manufacturing process to consistently deliver the intended performance of the product” (4). Clearly, gaining information needed to establish a design space, specifications, and manufacturing controls is an ongoing process. It begins with development-studies and continues during manufacturing throughout a product’s life cycle. The guideline communicates regulatory expectations with regard to how QbD enhances a sponsor’s understanding of product performance and knowledge of a process over a wide range of control parameters and material attributes. That should allow for flexibility in regulatory-review approaches of risk-based decisions and process improvements; it might also allow for acceptance of real time release testing. The goal is to facilitate submissions and their regulatory evaluation through an enhanced, science-based understanding such as that gained from DoEs and PAT as well as prior knowledge.
As is typical of regulatory guidelines, the specifics of “how” (which specific information, how much, and at which specific development stage) are left to sponsor companies to define and justify. Circumstances will vary greatly from company to company and even within larger companies as well as across different drug products/substances (DP/DS) and manufacturing process areas: active pharmaceutical ingredient (API), final product manufacturing (FPM) analytical methods, and so on.
Outsourcing
What aspects of working with a contract development and manufacturing organization (CDMO) can slow down upstream process development — and how can sponsors mitigate those problems? Many company-specific drivers affect the decision whether to outsource, ranging from availability of internal resources (e.g., skilled personnel, equipment, and facilities) to increased process complexity, process-control capability, and the need to obtain data/information quickly. Those making this decision also should consider the landscape of qualified CDMO partners to choose from. Many decision factors remain the same regardless of the development area (e.g., up-/downstream), but choices made in upstream process development can compound downstream from there over time. That is especially true when establishing long-term relationships typical with larger “one-stop shop” CDMOs that provide needed services and solutions throughout the development phases.
Contracted CDMOs are expected to deliver development and manufacturing milestones and goals on time, consistently, and at a reasonable cost — while meeting all quality requirements. The best way to mitigate potential problems down the road is doing your best “homework” on candidate CDMOs using tools such as questionnaires, site visits, meetings with company representatives, relevant web pages, and recommendations from sources you can trust.
Attributes to look for can be either “soft” (perceived) or “hard” (data based). A key soft attribute to consider is a CDMO’s cultural fit: its alignment with your organization’s core values, especially with regard to quality. A mutual understanding should come from open and clear communications between members and across levels at both companies. Another soft attribute is a CDMO’s reputation within the industry, and one way to determine this is by looking into its history with regulatory agencies — the US Food and Drug Administration’s (FDA’s) website being particularly transparent. Peer companies’ recommendations represent another source.
Hard attributes center on quality as well. The candidate organization should have a track record of experience in your area/s of need as well as the capacity and ability to match your evolving and growing needs as a product moves along phases from development to clinical to commercial. A diversity of technical expertise and service offerings also is important. Some companies have developed practices (such as holding “supplier days”) and models for weighing and ranking both soft- and hard-attributes information collected to facilitate decision-making on first-choice and backup CDMOs (5). Keep in mind that the partnership could be a long-lasting one. As with any type of relationship, maintaining one that works is priceless. It also saves a lot of time seeking for alternatives.
Characterization
How do analytics and early product-characterization efforts — e.g., host-cell protein (HCP) profiles and product-variant analysis — help move upstream process development along? Product characterization is at the core of CMC regulatory filings and is conducted throughout the course of drug development: from discovery through preclinical and clinical testing, then throughout the commercial life cycle. Thus, suitable analytical methods must be developed and validated to ensure safety, purity, potency, effectiveness, and consistency among batches. CMC expectations are described in guidelines such as those published by the FDA (6) and ICH (7–9). Assay suitability should be based on the nature of a DS — whether it is a monoclonal antibody, gene, or cell therapy, for example.
A key challenge of developing assays during process development is that of the “moving target.” Being in development means that a product is not yet final. Obviously, product-variant analytical methods cannot be finalized and fixed while both process and product are still in development. Likewise, HCP profiles cannot be defined fully until a production host cell has been selected, a bank generated, and a production process locked in. That said, beginning characterization (ideally leveraging HT assays) early in the development process is not only possible; it’s also beneficial. It provides early feedback into product development, especially with regard to required/desired PQAs.
Consider clone selection and cell-line development, which traditionally focused on cell growth and cell-specific productivity outputs. Yet the fastest growing and highest producing cells may not be the most stable — or those that meet target product-quality profiles best. That’s where early characterization (even applying assays that are not yet locked in) can provide a huge benefit in enabling decisions to be made earlier than otherwise and potentially saving valuable development time. Selecting a cell line based on meeting product-quality targets through analytical characterization, as well as on the basis of traditional outputs such as productivity and stability, would help to ensure selection of the best clone and help prevent the need to repeat rounds of selection — ultimately saving valuable development time. Moreover, obtaining characterization information early in development provides a head start toward addressing issues that require further optimization (on the assay or process side, or both), again providing the opportunity to save development time.
The utility of obtaining early product characterization information goes beyond cell-line development and extends to other aspects of upstream process development: media development, process optimization, CQA determination, and understanding the relationship between PQAs (e.g., a drug molecule’s physiochemical profile, structure, heterogeneity and biological activity) and clinical performance. This allows developers to make informed decisions that would accelerate development and reduce risk: such as in deciding which assays are most appropriate for lot release and stability testing during clinical trials and commercial manufacturing, including comparability studies following changes in manufacturing. Future work would focus on key enablers of assay adoption for routine product characterization early in product development: e.g., throughput/automation, sample availability, and cost reduction.
Caveats
When quality and safety are at stake, it can be a mistake to focus only on speed. How might you caution others when it comes to shortening upstream process development timelines? Because quality and safety never should be compromised (for speed, cost, or anything else), such considerations should be integrated into process/product development as early as is feasible. As discussed above, an in-depth understanding of product quality and process design calls for comprehensive product characterization, which is achieved through a wide spectrum of analytical methods using advanced instrumentation and relying on broad expertise in method development and interpretation capabilities.
Understanding of quality and safety is gained on the basis of sound science and quality risk management (QRM) principles as delineated in QbD principles by ICH Q8(R2) (4) and ICH Q9 (10). These principles have been promoted successfully by the FDA, the European Medicines Agency (EMA), and other regulators for about two decades, during which they have sped a number of regulatory approvals. The afforded higher level of product and process understanding facilitates success in development and ultimately reduces timelines. Examples include increased accuracy in predicting scale-up effects, reduced product variability, improved understanding of failure causes, and facilitated implementation of newer technologies.
The benefits of QbD continue postapproval throughout a product’s manufacturing life cycle. Increased process robustness together with expected reductions in deviation rates and failed batches will benefit drug manufacturers and patients alike.
References
1 Whitford WG. Single-Use Perfusion Culture Enables Continuous Bioproduction. Cell Culture Dish 23 July 2014; https://cellculturedish.com/single-use-perfusion-culture-enables-continuous-bioproduction.
2 Shimoni Y, et al. Development of a Novel Rolling-Tube Cell Culture Platform and Demonstration of System Feasibility. BioPharm Int. 23(8) 2010: 28–37; https://bioprocessintl.com/upstream-processing/bioreactors/qualification-of-scale-down-bioreactors-351594.
3 Shimoni Y, et al. Qualification of Scale-Down Bioreactors for Validation of Process Changes, Part 1 — Concept. BioProcess Int. 12(5) 2014: 38–45; https://bioprocessintl.com/upstream-processing/bioreactors/qualification-of-scale-down-bioreactors-351594.
4 ICH Q8(R2): Pharmaceutical Development. International Council for Harmonisation of Technical Requirements for Pharmaceuticals for Human Use, 2009; https://database.ich.org/sites/default/files/Q8_R2_Guideline.pdf.
5 Shimoni Y, et al. A Risk-Based Approach to Supplier and Raw Materials Management. BioProcess Int. 13(10) 2015: 10–15; https://bioprocessintl.com/upstream-processing/biochemicals-raw-materials/a-risk-based-
approach-to-supplier-and-raw-materials-management.
6 CDER. INDs for Phase 2 and Phase 3 Studies: Chemistry, Manufacturing, and Controls Information — Guidance for Industry. US Food and Drug Administration: Rockville, MD, May 2003; https://www.fda.gov/media/70822/download.
7 Q2(R1): Validation of Analytical Procedures. International Council for Harmonisation of Technical Requirements for Pharmaceuticals for Human Use, 27 October 1994, updated 2005; https://database.ich.org/sites/default/files/Q2%28R1%29%20Guideline.pdf.
8 Q3C(R6): Residual Solvents. International Council for Harmonisation of Technical Requirements for Pharmaceuticals for Human Use, 20 October 2015; https://database.ich.org/sites/default/files/Q3C-R6_Guideline_ErrorCorrection_2019_0410_0.pdf.
9 Q6A: Specifications — Test Procedures and Acceptance Criteria for New Drug Substances and New Drug Products — Chemical Substances. International Council for Harmonisation of Technical Requirements for Pharmaceuticals for Human Use: 6 October 1999; https://database.ich.org/sites/default/files/Q6A%20Guideline.pdf.
10 ICH Q9: Quality Risk Management. International Council for Harmonisation of Technical Requirements for Pharmaceuticals for Human Use, 9 November 2005, updated September 2015; https://database.ich.org/sites/default/files/Q9%20Guideline.pdf.
Yuval Shimoni is a principal specialist in quality assurance at Bayer HealthCare LLC, 800 Dwight Way, Berkeley, CA; 1-510-705-5775; [email protected]. The views and opinions expressed in this article are those of the author and do not necessarily reflect the official policy or position of any other agency, organization, employer, or company.