Smart, Good Manufacturing Practice Production of Cell and Gene Therapies: A Vision for the Future
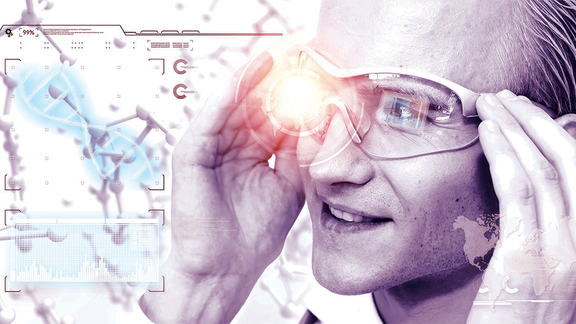
By the end of 2023, six gene-modified cell therapy products had received regulatory approval for treatment of aggressive B-cell lymphomas, mantle-cell lymphomas, B-cell acute lymphoblastic leukemia, and most recently, multiple myeloma. Such personalized medicines leverage patient-derived T cells that are genetically engineered to express chimeric antigen receptors (CARs) for recognition of a specific tumor antigen (e.g., CD19 proteins). Engineered cells are expanded and then are reinfused back into the patient as a CAR T-cell therapy. Building on the success of CAR-T as a modality, more than 2,000 clinical trials remain active worldwide for CAR-T candidates, including more than 200 programs in phase 3 studies (1).
However, access to such life-saving therapies remains limited. Although treatment costs represent a major barrier, another factor is that current cell-therapy manufacturing processes cannot meet the high market demand for such products. Healthcare analysts estimate that the global patient population with relapsed- and/or refractory-stage diseases that could be addressed with CAR-T cell therapies was 450,000 in 2019; that number is projected to exceed two million by 2029 as CAR-T moves into incrementally earlier lines of treatment (2).
Based on internal data, we estimate that manufacturing for all six therapies with US Food and Drug Administration (FDA) approval provided enough material to treat ~8,750 patients in 2023, whereas the approximate number of patients diagnosed worldwide with B-cell lymphoma (544,000), B-cell leukemia (64,190), and multiple myeloma (176,404) exceeded 750,000 (3–5). And in a 2022 clinical trial of heavily pretreated multiple myeloma patients, around 40% of patients needed to wait a year to receive Abecma (idecabtagene vicleucel). Unfortunately, ~25% of patients died while waiting for treatment (6).
The shortfall in treating the CAR-T addressable patient population stems, in large part, from limited production capability and/or capacity for such therapies. Our focus herein is to show how cell and gene therapy (CGT) companies can apply automation to overcome manufacturing bottlenecks and accelerate the availability of safe but economical treatments.
Commercial CAR-T manufacturing comprises several unit operations, each with distinctive equipment requirements. Yet manufacturing must occur on a short schedule (~10–20 days). Manual processes involve inherent risks for operator errors, which can impede production, trigger lengthy investigations, and prevent timely product release to the clinic. Automation can help improve regulatory compliance and product consistency by minimizing human errors during repetitive and cumbersome tasks.
Allogeneic products are manufactured in larger batch sizes than are autologous therapies, which are produced on demand for single patients. Therefore, allogeneic processes can use larger-scale equipment that lends itself well to process automation and standardization, whereas autologous therapies often require specialized equipment that operates at small scales. Although small-batch CAR-T processing enables parallel operation of multiple units in a manufacturing suite, such equipment tends to have limited automation capability and sometimes requires software that raises concerns for good manufacturing practice (GMP) compliance (e.g., with Title 21, US Code of Federal Regulations, Part 11). Because process equipment and analytical instrumentation are not always designed for automation, end-to-end automation of stepwise autologous-therapy manufacturing remains challenging.
Fully Automated Systems
Systems such as Lonza’s Cocoon cell-therapy manufacturing technology and Miltenyi Biotec’s CliniMACS Prodigy platform offer significant automation for process steps in several autologous protocols, including those for CAR-T, tumor-infiltrating lymphocytes (TILs), and mesenchymal stem cells (MSCs). But market adoption of automation still lags.
One factor is that all-in-one systems raise novel concerns for operating in a GMP space because such technologies are difficult to validate and maintain. By design, autologous therapies are highly time sensitive: “Vein-to-vein” time (from collection of patient blood to therapy administration) factors critically into patient outcomes. However, fully automated systems still require skilled staff to troubleshoot process interruptions — and perhaps have system suppliers on speed dial to address manufacturing issues. Therefore, companies might be cautious in implementing highly automated systems early in product development, when scientists are trying to define process designs and parameters based on highly variable patient-derived cells as starting material. For example, equipment setups such as the CliniMACS Prodigy platform do not enable operators to make timely process corrections without contacting system vendors. Updated firmware could allow for such changes, but equipment models with such capabilities are not yet prevalent.
Troubleshooting currently necessitates close coordination between CGT developers and equipment vendors to resolve error logs quickly, perform real-time problem-solving, and return interrupted production processes back to normal operation. That is especially critical when processes run overnight for multiple days: Device troubleshooting that must occur outside of typical work hours depends on timely vendor support for error resolution.
Another concern is that intellectual property (IP) concerns from equipment vendors can complicate manufacturing, resulting in process hold-ups and batch failures, which are unacceptable in the autologous therapy space. Collaboration between system vendors and CGT developers can help to establish common ground and safeguard IP concerns while ensuring operational agility. Besides speeding up processes, such partnerships also could reduce barriers to future equipment adoption.
Staffing Facilities for Automated Operations
Current staffing needs for CAR-T manufacturing are threefold. First, the industry must expand opportunities for education on highly technical manufacturing processes. At a minimum, operators must complete community-college–level coursework with specialized training for GMP cleanroom operations, cell-culture principles, and application of automated equipment systems. The North Carolina Biotechnology Center provides one such training program. Table 1 lists some universities with examples of similar training offerings. Vendor-sponsored courses that are equipment specific also could help to fill the CGT industry’s need for operators with specialized knowledge and skills.
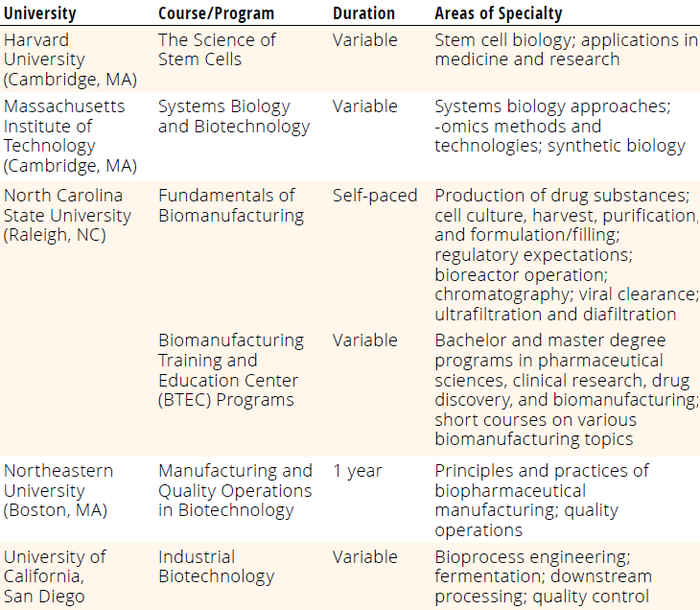
Table 1: Examples of specialized training in biomanufacturing and biotechnology from US universities; for more detailed information about the programs below, refer to the respective university websites or contact their biotechnology departments.
Qualified engineering and maintenance teams are essential to servicing automated biotechnology equipment. CGT companies must support such staff with robust, in-house training and refreshers from equipment vendors. Training should include detailed standard operating procedures (SOPs) and procedures for batch records.
For qualification and validation, CGT companies also need skilled technical staff who can perform installation, operational, and performance qualification (IQ/OQ/PQ) of complex equipment and instruments. Some developers might contract this service out to vendors or consultants.
Plant Digitalization
Automation can reduce autologous manufacturing costs significantly while improving regulatory compliance and increasing agility on the manufacturing floor. CAR T-cell therapies currently cost US$350,000–450,000 per dose. In manual production processes, human errors can trigger lengthy quality investigations, which increase both cycle time and cost. CGT companies could explore “smart” technologies — e.g., smart glasses — that can be programmed to give real-time, step-by-step guidance to production and maintenance staff. With such technologies, operators can document completion of process steps through simple voice commands, and dictations can be captured in electronic batch records that are stored locally or in a cloud infrastructure. Cloud-based manufacturing execution system (MES) software with step-by-step instructions integrated into connected devices such as tablets and smart glasses could improve process execution within a GMP space.
Industrial-grade smart glasses have come a long way since the launch of Google Glass technology in July 2017. Newer models are more sophisticated and have significantly better processing power, memory storage, network connectivity, battery life, form factors, and usability (Table 2). Some models can be sanitized with harsh detergents (e.g., Spor-Klenz sterilant, Steris) and disinfected with vaporized hydrogen peroxide (VHP) for use in cleanroom operations. Such units are available as zone-1–compliant (intrinsically safe, class I, division 1) technologies for environments requiring explosive atmosphere (ATEX) certification.
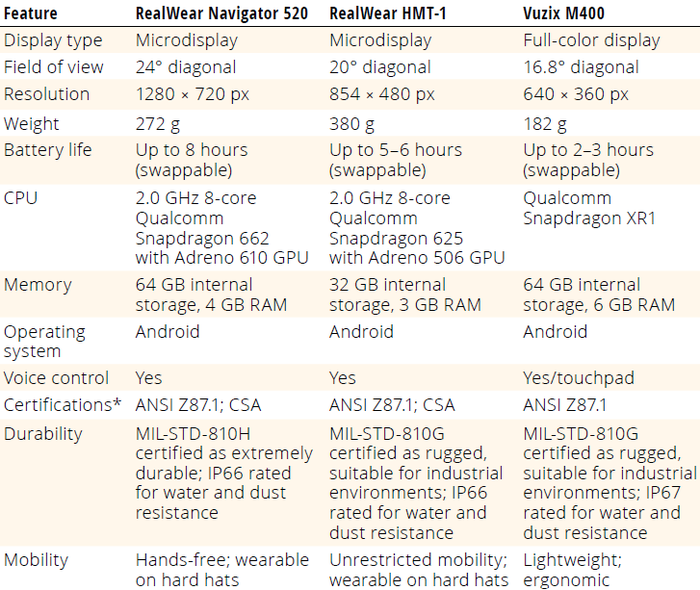
Table 2: Specifications of three commercially available, industrial-grade smart glasses.
ANSI = American National Standards Institute; CPU = central processing unit; CSA = CSA Group Standards
Organization; GPU = graphics processing unit; IP = ingress-protection code; MIL-STD = US Department of
Defense Test Method Standard, Environmental Engineering Considerations, and Laboratory Tests;
RAM = random-access memory
* ANSI Z87.1 is a certification for safety glasses, not for smart devices. Smart-glass devices are monocular and
can fit over (or be connected to) different types of safety glasses/goggles but are distinct from those glasses.
Meanwhile, momentum continues to build in the pharmaceutical and biotechnology industries to adopt an operator-centered model in which mobile devices provide tools and information needed for process execution, especially when systems can integrate directly with SAP, Aveva PI (formerly OSIsoft Pi), DeltaV, and other existing enterprise systems.
CAR-T manufacturers should consider using such devices to improve compliance costs and speed to market. We predict that, with the advent of artificial intelligence (AI) and its increasing integration into software, powerful wearables will reach the hands of operators in short order. Those technologies could provide operators with AI-generated instructions, real-time data analysis, “computer vision” or multimodal analysis, and safety reminders while performing tasks.
The greatest challenge for smart biomanufacturing technologies will lie in how vendors and users navigate regulations for data generation, quality, storage, retrieval, distribution, access, and validation. A consortium with members representing biotechnology-industry leaders and equipment vendors and with input from regulatory agencies could help to formulate best practices for design and use of such equipment and systems.
Integrating peripheral devices (e.g., smart glasses) with enterprise systems could enable operators to navigate electronic batch records with ease through digitally guided workflows. That would reduce use of paper records and keyboards in cleanroom settings. Peripheral devices also would help to reduce operator fatigue and distractions, which are major reasons for errors in high-risk manufacturing processes. For instance, operators might receive safety information or audio/visual reminders for frequently missed process steps.
Such technologies also provide means for capturing deviations contemporaneously with photographs, video recordings, or even live streaming for quality review and troubleshooting. Some CGT developers have leveraged the live-streaming capabilities of smart glasses to great effect, performing process simulations and conducting remote observation of contract development and manufacturing organizations (CDMOs) as they worked with client materials.
Automating Documentation
Documentation demands in cleanrooms have become increasingly onerous with the need to capture detailed experimental information accurately and contemporaneously. Operators often record data related to GMP-process execution in a dedicated computer kiosk, which might be far from the work area. That would require operators to pause after each process step to enter data manually. Data capture through voice commands and integrated equipment systems would ease such burdens while decreasing operator fatigue and movement in cleanroom spaces. Some companies staff cleanroom areas with an additional operator equipped with a laptop for contemporaneous data capture and documentation, but the added operator increases contamination risks.
Smart glasses could send encrypted process-execution data to platforms through a secure wireless-fidelity
(Wi-Fi) network or cellular connection. For such technologies to be useful, companies must establish robust Wi-Fi infrastructure during design of GMP spaces. Poor digital connectivity is a common issue when cleanroom facilities are not well planned or are maintained insufficiently for automation. Smart peripheral devices also can scan quick-response (QR) codes on equipment and paperwork, material and equipment barcodes, and Bluetooth beacons, enabling integration with equipment systems for sensor-data entry.
Using smart technologies, operators can connect on demand with support staff outside a cleanroom space. Live broadcasts from cleanrooms to supervisory/quality staff could help to prevent process deviations, batch failures, and ultimately, loss of drug products for patients. Such capabilities also can provide managers with needed clarification before making potentially costly decisions. A live-broadcast setup also enables remote two-person verification, in which reviewers can remotely observe an operator’s execution of a step in a procedure and then provide an electronic signature as a second verifier for that step in the batch record. A remote second-person verifier could review multiple operators simultaneously in different facilities globally, enabling gowned operators in the facility to focus on their tasks without needing to be pulled away for in-person verification.
The operations model described above provides for rapid technical support, quality oversight, and operator verification of steps performed. Peripheral devices represent powerful cleanroom-automation tools with a compelling case for increased adoption in time-sensitive manufacturing contexts, including that for producing autologous therapies.
The Future of CAR-T Manufacturing Automation
The future of CAR-T and other CGTs will depend on how quickly the industry moves toward a highly integrated, automated, and digitalized approach to manufacturing. Biotechnology developers, CDMOs, and point-of-care manufacturing and delivery centers should strive for a connected-manufacturing future, in which data sharing and collaboration are embedded into each process and thus become a normal way of working rather than an afterthought. As cost pressures on therapies increase, technologies for automation, small-scale autologous processing (e.g., manufacturing-in-a-box systems), and large-scale allogeneic manufacturing will become increasingly intertwined with advanced MESs, cloud computing, AI, and edge computing, by which smart devices that are proximal to manufacturing processes provide advanced controls over operations.
Such automation will help foster more streamlined, responsive, and flexible CGT-manufacturing systems than are currently available, breaking down barriers to accessibility of life-changing medicines. For instance, connecting automation with advanced MESs could yield CGT products of the highest possible quality with fast turnaround times while bringing production processes close to the point of care. Ultimately, CGT developers will be able to manufacture CAR-T and related therapies with small-scale, automated systems in hospitals, enabling direct delivery of personalized treatments to patients.
The journey toward such a future undoubtedly will be challenging, yet the potential reward is immense, particularly for meeting demand from large patient bases. That is especially true considering potential expansion of applications for CAR-T and related therapies, which now are in clinical trials for autoimmune disorders such as multiple sclerosis (6–8). With commitment, innovation, and collaboration, the industry can unlock CGT’s potential for broad patient care using cost-efficient, agile, and fully compliant manufacturing processes.
References
1 Hunt T. 2023 Industry Update at the Cell and Gene State of the Industry Briefing. Alliance for Regenerative Medicine: Washington, DC, 9 January 2023; https://www.youtube.com/watch?v=-Ga5bbUGIbY.
2 Mikulic M. Estimated Addressable Patient Population for CAR-T Cell Therapies Worldwide as of 2019. Statista 19 April 2022; https://www.statista.com/statistics/1098463/global-addressable-patient-population-car-t-cell-therapies-estimate.
3 Mafra A, et al. Global Patterns of Non-Hodgkin Lymphoma in 2020. Int. J. Cancer 151(9) 2022: 1474–1481; https://doi.org/10.1002/ijc.34163.
4 Yi M, et al. Global Burden and Trend of Acute Lymphoblastic Leukemia from 1990 to 2017. Aging 12(22) 2020: 22869-22891; https://doi.org/10.18632/aging.103982.
5 Huang J, et al. The Epidemiological Landscape of Multiple Myeloma: A Global Cancer Registry Estimate of Disease Burden, Risk Factors, and Temporal Trends. Lancet Haematol. 9(9) 2022: e670–e677; https://doi.org/10.1016/s2352-3026(22)00165-x.
6 Mullard A. CAR-T Therapy for Multiple Sclerosis Enters US Trials for First Time. Nature 22 February 2024; https://doi.org/10.1038/d41586-024-00470-5.
7 NCT06138132. A Study of Anti-CD19 Chimeric Antigen Receptor T-Cell (CAR-T) Therapy in Subjects with Non-Relapsing and Progressive Forms of Multiple Sclerosis. ClinicalTrials.gov 30 April 2024; https://classic.clinicaltrials.gov/ct2/show/NCT06138132.
8 Walton C, et al. Rising Prevalence of Multiple Sclerosis Worldwide: Insights from the Atlas of MS, Third Edition. Multiple Sclerosis J. 26(14) 2020: 1816–1821; https://doi.org/10.1177/1352458520970841.
Sridhar Venkatesan, BPharm, MSc, MS, is a senior consultant; James Beltzer, PhD, is a principal consultant; and Joseph Rininger, PhD, is a managing director, all at Latham Biopharm Group (part of Sia Partners), 6810 Deerpath Road, Suite 405, Elkridge, MD 21075; https://www.lathambiopharm.com. Frank Maggiore is director of strategy, key account customer success manager, and chief futurist at Apprentice.io,101 Hudson Street, 38th Floor, Jersey City, NJ 07302. Please email inquiries to Lauren Schoukroun-Barnes, PhD, PMP, CSCP, RAC; [email protected].
You May Also Like