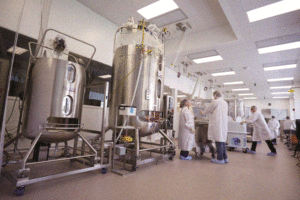
With product portfolios that cover both the upstream (production) and downstream (processing) aspects of biopharmaceutical manufacturing, large single-use technology suppliers such as GE Healthcare can address questions of integration and continuous processing with a holistic perspective. (WWW.GELIFESCIENCES.COM)
Despite decades of advancement in characterization analytics, biotherapeutics still are largely defined by the manufacturing processes used to make them. This linking of process to clinical results (and thus to commercial success) has made the biopharmaceutical industry somewhat risk-averse when it comes to the adoption of new technologies. That desire to “derisk” biomanufacturing through better process understanding — as well as the need to adapt to uncertainties in patient population size through process flexibility — in turn drives the need for adoption of new technologies.
Case in point: continuous processing. It’s pretty much a “given” in many other industries — such as those making chemicals and food products — and even fairly established in the larger pharmaceutical industry that makes synthetic small-molecule drugs. But the concept has only just begun to make inroads with biomanufacturers, who have until recently worked mainly in batch or fed-batch mode. Single-use technologies have largely enabled them to consider the possibility of process intensification and going continuous. And BPI has documented this increasing interest over the past few years (1–21).
Continuous Upstream Production
Until recently, perfusion culture was used in biomanufacturing almost exclusively for difficult-to-express (low-titer) proteins and those known to be sensitive to culture conditions. Batch/fed-batch processes could produce them, but by the time a culture was over, most of the early expressed proteins would have deteriorated beyond retrieval. Genzyme (Sanofi) is one company that, as a result, has amassed quite a lot of experience with continuous culture — which importantly includes continuous harvest.
Perfusion culture allows for high-density cultures that maximize productivity in relatively small bioreactors. It requires continual feeding — and thus uses up large volumes of culture media/supplements — as well as continuous removal of metabolites and product, with dilution rates exceeding the cell growth rate. This requires a means of retaining cells in a bioreactor while removing (and replacing) the supernatant around them. Hollow-fiber reactors offer one solution, with new technological advancements addressing their problems of old. The article by Steve Waniger in this featured report goes into some detail about this approach.
Other companies, meanwhile, have focused on ways to enable continuous culture in traditional bioreactors (whether stainless steel or single-use). One example is Repligen’s XCell alternating tangential-flow (ATF) system, originally developed by Refine Technology (5). It integrates a diaphragm pump, a stainless steel housing, and a single-use hollow-fiber filter cartridge for nearly linear scale-up of operation. Unique flow dynamics prevent the kind of filter failure that typically comes with separation of complex suspensions that feature high bioburden levels.
Continuous Downstream Processing
Clearly, the primary difficulty in making biomanufacturing continuous comes from the products of metabolism. Process engineers are faced essentially with the need to replicate true biological organisms, which use circulatory systems to bring in nutrients and remove waste. That sounds straightforward enough, but it took biology millions of years to perfect the concept. And but for the seemingly immortal lobster and incredibly hardy tardigrade, every organism does die eventually!
After harvest — which in continuous production processes goes on indefinitely — the process stream that begins with supernatant must be refined down to purified drug substance that’s ready for formulating into a drug product. Host cells don’t just express the desired protein but also many others. Dead cells become debris, including nucleic acids and potential pathogens. Viruses and bacteria must be assumed to accompany all that, and steps must be taken to remove them. And the nature of all these things is so varied that no single separation technology can handle them all.
Most downstream processes that have become familiar to biopharmaceutical companies and their government regulators are batch processes: centrifugation, depth and tangential-flow filtration, chromatography, virus inactivation. Going continuous with these methods has been — and remains — a challenge (20). But as Margit Holzer explains in her contribution to this featured report, continuous options are in development or available for most of them now. Again, single-use technologies seem to be key to making it all possible.
A Case Study
Despite the well-reported high cost of product capture chromatography using expensive resins such as protein A, cost of goods (CoG) in bioprocessing has been a low-priority issues for most biopharmaceutical companies. Until only recently, the biomanufacturing process defined these products and determined their safety and efficacy.
Generating as deep an understanding of how that process affects such molecules in as early a stage as possible is critical for accelerating safe and efficacious products into the clinic and beyond. GlaxoSmithKline (GSK) uses a “one-stream approach,” observing manufacturing processes holistically to optimize each unit operation with all other units in mind for the best overall output. The more each stage is understood through rapid and reliable process characterization, the better will be the overall process and the lower will be its CoG.
Many industry experts estimate that only ~30% of biotherapeutic CoG comes down to true direct and indirect costs (consumables, labor, and operational overhead). The remaining ~70% is attributable to amortization of the installed facility base. Naturally, this should draw financial focus toward maximizing facility output — both for installed and future facilities. Holistic facility productivity and the components that enable it are therefore major drivers for the biopharmaceutical industry today. GSK has several initiatives in place to look ahead and develop a way to meet essential productivity increases.
One example is its collaboration with the venture-backed UK company Puridify. Their work was recognized with the “best industrial collaboration” award at the 2016 International Awards, where Puridify’s FibroSelect technology also was a finalist for “best downstream technology.” It is a single-use protein A alternative to the porous-bead packed beds that have been the industry standard for at least 15 years of MAb manufacturing. The supplier’s goal is to change as little as possible while offering processing advances that the industry demands.
Traditional single-column protein A capture stages offer productivities of ~6-12 grams of purified MAb per liter of adsorbent per hour processed (g/L/h). Puridify’s adsorbent technology uses the same chemical base materials and process infrastructure to deliver a 50-fold productivity improvement while maintaining product critical quality attributes (CQAs). Mechanically strong cellulosic nanofibers exhibit high surface areas and an open, porous fixed structure. Functionalization of that surface for protein adsorption produces materials with very high capacities at flowrates >300 membrane volumes each minute. The functional surface area becomes immediately accessible to the mobile phase, which decouples mass transfer from flow rate, relying on residence times of under a second.
With rapid cycling in a good manufacturing practice (GMP) environment for an equivalent bioreactor harvest, users can get the same results from a nanofiber adsorbent cartridge that is significantly smaller than the column equivalent. The resulting process unit can be sized such that its lifetime (in cycles) can be exhausted by one batch, making single-use operation economically feasible.
Shrinking unit operations fits with a trend in the industry toward smaller, modular, multiproduct facility design. This new way of operating the protein A step could offer greater flexibility in designing processes to be carried out in a fraction of the time of current purification — or in the same time but with a 50× smaller unit that can be discarded immediately after a batch is processed. Users can choose any point between those two extremes.
Product capture chromatography — in particular using protein A affinity resins — has been crucial in process development and manufacture of MAb therapeutics over the past 20 years. As such, chromatography is a step that requires much process development time spent in getting packed beds to perform to their maximum capability. Most optimization effort stems from limitations inherent to the media and packing: e.g., mass-transfer and pressure-drop limitations, channeling, and bead-wall support effects. Thus, limited throughput has prevented this unit operation from being economically accessible in a single-use format.
The goal is not simply to reduce CoG associated with chromatography, but rather to enable new overall processing strategies to give biomanufacturers greater flexibility in their choice of operations. That should maximize the productivity of new and existing drug manufacturing facilities. The nanofiber technology continues to be evaluated by other biopharmaceutical companies and has already demonstrated productivities of 500 g/L/h with its high flow rates and capacities.
Bridging the Gap Between Upstream and Downstream: A further part of this study is to consider other processes in which high-throughput protein purification could be of value (e.g., in lead-candidate cell-line selection, when time and costs of development are critical factors). GSK tested it along with 48 ambr 15 bioreactors (Sartorius Stedim Biotech) for a rapid and reliable process. Investigators built an automated workflow that loaded unfiltered supernatant onto FibroSelect protein A high-throughput screening cassettes using a Tecan liquid-handling platform. Purified MAb was then collected in a single-well of a 96-well microplate. A single cycle purified 600 µg of the target protein from each of the 48 individual bioreactors in under two hours of total processing time, providing material needed for subsequent analytical steps. The single-use modality of the membrane cassettes negated the need for a filtration step between them and the bioreactor. This demonstrates an enabling technology for cell-line development that removes the downstream bottleneck in processing a large number of individual feed streams.
Puridify is further developing the ability to purify less-clarified feed streams at larger, industrially relevant scales. The company expects to evaluate such operations as a further part of the GSK collaboration in 2017. Potentially eliminating an entire unit operation from bioprocessing — without introducing secondary implications such as those related to viral clearance — is a proven means of cost reduction and productivity enhancement.
Bringing It All Together
Reading the above, you might wonder whether those nanofiber cartridges could be installed downstream of perfusion reactors for continuous harvest/capture operations — interrupted only occasionally when one cartridge has reached its limits and needs to be replaced. These are the kinds of ideas that single-use and other technological innovations in bioprocessing are allowing process engineers to consider. The means by which they do so can resemble the modeling method described in the article following this one.
For continuous processing to work, downstream technologies must be applied further upstream than ever before. Does this suggest that the wall between production and processing ultimately could disappear? In a practical sense, perhaps: With closed systems, they could happen all in the same room. But from a scientific standpoint, their basic natures remain distinct. And contamination concerns are likely to keep upstream and downstream apart in biomanufacturing facilities for some time. Biological entities become ill when such things go awry, and you might need a day or two off work to deal with a severe bout of food poisoning. But a “sick” bioprocess can end up costing its host company (and the patients served by that company’s products) a lot more.
References
1 Bisschops M, et al. Single-Use, Continuous-Countercurrent, Multicolumn Chromatography. BioProcess Int. 7(6) 2009: S18–S23.
2 Li L, et al. A Single-Use, Scalable Perfusion Bioreactor System. BioProcess Int. 7(6) 2009: 46–54.
3 Whitford WG. Cadwell JJS. Interest in Hollow-Fiber Perfusion Bioreactors Is Growing. BioProcess Int. 7(9) 2009: 54–63.
4 Acuna J, et al. Modeling Perfusion Processes in Biopharmaceutical Production. BioProcess Int. 9(2) 2011: 52–58.
5 Bonham-Carter J, Shevitz J. A Brief History of Perfusion Biomanufacturing: How High-Concentration Cultures Will Characterize the Factory of the Future. BioProcess Int. 9(9) 2011: 24–30.
6 Langer ES. Trends in Perfusion Bioreactors: The Next Revolution in Bioprocessing? BioProcess Int. 9(10) 2011: 18–22.
7 Klykov SP, Kurakov VV. A New Kinetic Structured Model for Cell Cultivation in a Chemostat. BioProcess Int. 10(9) 2012: 36–43.
8 Rios M. Developing an Integrated Continuous Bioprocessing Platform. BioProcess Int. 10(11) 2012: S30–S33.
9 Whitford WG. Supporting Continuous Processing with Advanced Single-Use Technologies. BioProcess Int. 11(4) 2013: S46–S52.
10 Whitford WG, Cadwell JJS, Hardy JC. Single-Use, Continuous Processing of Primary Stem Cells. BioProcess Int. 12(3) 2014: S26–S33.
11 Janas ML, et al. Perfusion’s Role in Maintenance of High-Density T-Cell Cultures. BioProcess Int. 13(1) 2015: 18–26.
12 Benjamin Wright B, et al. A Novel Seed-Train Process: Using High-Density Cell Banking, a Disposable Bioreactor, and Perfusion Technologies. BioProcess Int. 13(3) 2015: S16–S25.
13 DiCesare C, et al. Development, Qualification, and Application of a Bioreactor Scale-Down Process: Modeling Large-Scale Microcarrier Perfusion Cell Culture. BioProcess Int. 14(1) 2016: 18–29.
14 Mothes B, et al. Accelerated, Seamless Antibody Purification: Process Intensification with Continuous Disposable Technology. BioProcess Int. 14(5) 2016: 34–58.
15 DePalma A. Special Report on Continuous Bioprocessing: Upstream, Downstream, Ready for Prime Time? BioProcess Int. 14(5) 2016: insert.
16 Monge M. Deciding on an Integrated Continuous Processing Approach: A Conference Report. BioProcess Int. 14(6) 2016: 67–68.
17 Fenge C, et al. Continuous Cell Culture Operation at 2,000-L Scale. BioProcess Int. 14(10) 2016: S22–S29.
18 Schmidt SR. Drivers, Opportunities, and Limits of Continuous Processing. BioProcess Int. 15(3) 2017: 30–37.
19 Willard SS, et al. Comparing Culture Methods in Monoclonal Antibody Production: Batch, Fed-Batch, and Perfusion. BioProcess Int. 15(3) 2017: 38–46.
20 Munk M. The Industry’s Hesitation to Adopt Continuous Bioprocessing: Recommendations for Deciding What, Where, and When to Implement. BioProcess Int. 15(4) 2017: 14–17.
21 Kenyon D, Jorjorian P. How to Set Up a Perfusion Process for Higher Productivity and Quality. BioProcess Int. 15(4) 2017: 48–52.
Cheryl Scott is cofounder and senior technical editor of BioProcess International, PO Box 70, Dexter, OR 97431; 1-646-957-8879; [email protected]. Oliver Hardick, PhD, is chief executive officer of Puridify Ltd., Stevenage Bioscience Catalyst, Stevenage, SG1 2FX United Kingdom; [email protected]. Will Lewis is head of biopharmaceutical purification research at GlaxoSmithKline.