The New Continuous and Controlled Approach to Nanoparticle Processing
Successful production of lipid nanoparticles (LNPs) is predicated on a thorough understanding of critical process parameters (CPPs) and establishment of highly controlled manufacturing processes. As demand for RNA-based therapeutics and vaccines grows — exemplified by the rapid advancement of mRNA COVID-19 vaccines — continuous manufacturing has emerged as a pivotal solution for attaining robust and scalable production of nucleic-acid LNPs. An adaptable end-to-end production platform can streamline nanoparticle generation and downstream operations within a single, closed system. That setup offers significant advantages, including smaller production footprints, reduced facility and storage needs, enhanced scalability, minimized human intervention, and superior particle-size control.
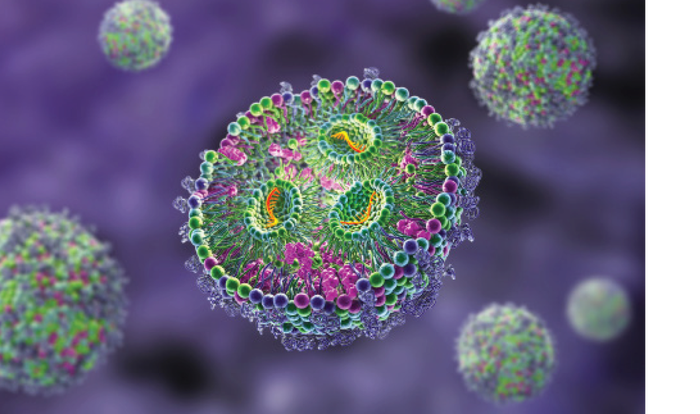
HTTPS://STOCK.ADOBE.COM
The Current Landscape of Nucleic-Acid Delivery and Processing
The advent of LNP formulations marked a significant milestone in the pharmaceutical industry. In 2018, Onpattro (patisiran), a treatment for hereditary transthyretin-mediated amyloidosis (hATTR), made history as the first nucleic-acid–based drug encapsulated in LNPs to receive US Food and Drug Administration (FDA) approval. Market authorization of mRNA-based COVID-19 vaccines from Pfizer/BioNTech and Moderna further highlighted the safety and efficacy of RNA therapeutics, creating momentum for continued research and development (R&D) in the field. Numerous clinical trials are underway examining various RNA-based therapeutics and vaccines as well as DNA products, gene-editing technologies based on clustered regularly interspaced short palindromic repeats (CRISPR), and oncology targets. Many such candidates rely on LNPs for their ability to stabilize nucleic acids and facilitate cellular entry, making them essential components in modern biopharmaceutical development. The size and shape of nanoparticles play crucial roles in determining their properties, which in turn influence drug performance and efficacy. Stable particles created from diverse source materials have emerged as fast-growing, beneficial technologies in recent years.
Traditionally, processing equipment does not provide for in-line or continuous control of critical quality attributes (CQAs), and quality analytics only give results after batch processing. That can lead to batch rejections and often does not address nanoparticle CQAs such as size, shape, surface charge and chemistry, drug-loading/encapsulation capacity and release kinetics, stability, biocompatibility, targeting ability, and so on.
Nanoparticle size can affect stability, biodistribution, and cellular uptake. Nanoparticles used in biomanufacturing are typically 10–100 nm in diameter. Relatively small nanoparticles have better cellular uptake and can penetrate tissues more effectively than larger ones can.
The shape of nanoparticles can influence their interactions with cells and tissues. Different shapes (e.g., spheres, rods, and discs) have specific cellular-uptake mechanisms and biodistribution profiles.
LNP surface charge often is determined by the presence of functional groups or coatings. It can affect particle stability, cellular uptake, and interaction with biological barriers. Positive surface charge can enhance cellular uptake; negative charge can improve particle stability.
Surface chemistry plays a crucial role in LNP interactions with biological systems. Functional groups and coatings on nanoparticle surfaces can enable specific targeting, enhance stability, or control drug release.
For drug-delivery nanoparticles, the ability to load and release therapeutic agents efficiently is critical. High drug-loading capacity helps to ensure effective delivery of therapeutic payloads to target sites. Nanoparticles should have a high-encapsulation capacity for drugs and other therapeutic agents, ensuring efficient delivery and maximum therapeutic effect.
Drug-release kinetics from nanoparticles can reduce or enhance therapeutic efficacy. Controlled drug-release over a desired period can optimize drug concentration at the target site and minimize off-target effects. Nanoparticles should have the ability to release drugs in a controlled manner, either in response to specific stimuli or over a prolonged period.
Nanoparticle stability is important to ensure integrity during storage and transportation. Stability can be influenced by factors such as aggregation, degradation, or changes in physicochemical properties over time.
Nanoparticles should be biocompatible to minimize adverse effects and ensure their safe use in vivo. Biocompatibility requirements include low toxicity, minimal immune response, and an absence of long-term adverse effects. Nanoparticles should have low toxicity to minimize adverse effects in the body.
Targeting ability determines how well LNPs can accumulate at the desired site of action, such as a tumor or inflamed tissue. Targeting ligands and functional groups on a nanoparticle’s surface can facilitate specific interactions with target cells or tissues.
Consistency in manufacturing and reproducibility of nanoparticle properties can ensure batch-to-batch consistency and reliable performance of nanoparticles for therapeutic applications.
Nanoparticles must respond to specific stimuli, (e.g., enzymes, pH, temperature, light, and magnetism) for targeted drug delivery and controlled release at the desired site.
Specific CQAs can vary depending on nanoparticle type and intended application. Different modalities, architecture, and charge all require attention to make nanoparticle delivery possible. Because size and shape play major roles, nanoparticle homogeneity is essential. Lack of control, limited throughput, and low yields are major challenges right now and will become a major focus as nanoparticle capacity demand increases.
Overcoming Challenges
Production of nanoparticles and LNPs is a complex undertaking because of the intricate interplay of several factors, including the CQAs of the nucleic acids and lipids used. The manufacturing process involves several critical stages, such as component mixing, solvent removal, surface modification of particles, and purification steps. Although those stages can be controlled in small-scale settings, ensuring consistency and reliability is difficult as scale increases. Real-time monitoring of vital process parameters can help to facilitate successful production scale-up. Implementation of robust processing controls is fundamental to the successful synthesis and manufacturing of nanoparticles for several other reasons as well.
Reproducibility: Consistency during production can help to ensure that each batch of nanoparticles meets predetermined specifications. Variability in components or processing conditions can lead to significant final-product differences, affecting performance and efficacy.
Quality Maintenance: Ensuring that nanoparticle quality remains high throughout the production cycle is critical. Control strategies allow for regular assessment of product attributes, helping to identify and mitigate deviations early on.
Safety Assurance: Establishing stringent processing controls minimizes potential risks associated with manufacturing, such as contamination and hazardous conditions, thereby safeguarding both the product and process operators.
Scalability: Effective processing controls facilitate transition from small-scale laboratory production to commercial manufacturing. By understanding and controlling key parameters, manufacturers can replicate conditions at scale to yield high-quality nanoparticles.
Regulatory Compliance: Adherence to guidelines requires comprehensive documentation of processes and product quality. Robust processing controls help companies to meet such regulatory requirements, ensuring that a manufacturing process is transparent and accountable.
Continuous manufacturing has emerged as a strategic solution to many challenges associated with LNP production. Compared with batch processing, such an approach is designed to optimize interactions among smaller quantities of materials for shorter time frames, which can result in improved lipid-based formulations. By minimizing the duration for which a drug remains nonencapsulated and reducing process complexity, controlled continuous manufacturing provides for tightened oversight over product quality and consistency. Moreover, the strategy diminishes the risks of both human error and contamination, which are more prevalent in traditional batch processes. Streamlining operational steps not only speeds production cycles, but also reduces holding times between process stages. By minimizing those intervals, companies can reduce the risk of destabilization and degradation of LNPs, ensuring that the final product retains its intended functionality and efficacy and supporting the successful transition of nanoparticle-based technologies into clinical and commercial applications.
Streamlining Production with Continuous Methodologies
Traditional batch processes for producing nucleic-acid LNPs typically involve six to nine distinct and multidimensional steps. Those often require significant time and resources, with each phase needing hold steps and extensive testing to ensure quality and compliance. Such a fragmented approach can lead to inefficiencies while prolonging overall production timelines. By contrast, continuous nanoparticle generation streamlines the entire process into a single, cohesive operation within a closed system. It eliminates the need for intermediate hold steps and reduces the frequency of testing between phases, minimizing waste and enhancing operational efficiency.
One advancement in continuous LNP production is the use of turbulent mixing technology, which facilitates processing at high flow rates while maintaining precision in particle formation. The technology ensures that generated nanoparticles are uniform in size and quality to safeguard their efficacy in therapeutic applications. Moreover, incorporation of real-time analytics into a continuous processing framework allows for midstream adjustments based on ongoing monitoring. That capability is crucial for maintaining product integrity, helping operators to identify and fix potential issues before they lead to batch failures — undesirable outcomes that result in significant material loss and increased production costs. Without robust process controls, manufacturers would be unable to assess product quality until batch completion. The advantages of continuous processing in LNP production are numerous and compelling. Continuous processes typically provide for increased production volumes and efficiencies, enabling manufacturers to meet rising demand without sacrificing quality. By eliminating unnecessary hold steps, waste is reduced significantly, promoting a more sustainable manufacturing approach. Continuous systems often require less physical space than traditional batch setups do, offering an attractive option for modern manufacturing facilities. The design of functionally closed systems minimizes exposure to external contaminants, improving purity levels in final products. And continuous processing relies on automated systems that require fewer operators, thus lowering labor costs and training requirements.
To achieve the full benefits of continuous-processing technologies, manufacturers must implement them alongside advanced in-line process analytics. That combination can provide significant efficiencies, reduce costs, and improve product quality. The complexity of LNP formation further complicates manufacturing, with many parameters — including nucleic-acid type, lipid surface charge, and steric stability — that must be managed. Understanding how lipid ratios influence nucleic-acid encapsulation is critical because those relationships can affect nanoparticle stability and performance. Therefore, it becomes imperative to develop precise formulations and refine continuous production processes to maintain control over CQAs, ensuring the successful application of LNPs in therapeutic contexts.
Future Innovations in LNP Production
A comprehensive, end-to-end manufacturing platform for nucleic-acid LNP products would leverage a closed system and integrate a robust suite of process analytical technologies (PATs), seamlessly combining particle generation with downstream operations, including buffer exchange, surface modification, purification, and fill–finish processes. That platform could use an ethanol-injection strategy complemented by single-pass tangential-flow filtration (TFF). That method would eliminate the need for recirculation of process solutions, reducing biofouling while enabling uninterrupted operations.
Continuous LNP-production systems yield high-quality nanoparticles with improved characteristics compared with traditional batch processes. In-line and at-line sensors continuously monitor key parameters such as temperature and pressure, ensuring an in-depth understanding of equipment performance and material flow to enhance process control. Additionally, the fully closed natures of such systems boost operational efficiency, allowing a single set of operators to oversee the entire process and thereby reducing the time and costs associated with workforce training and cleanroom upkeep.
In the future, the emphasis will not only be on refining existing processes but also on exploring the integration of fill–finish activities, which are currently hindered by lengthy regulatory testing timelines. By implementing innovative real-time monitoring solutions, manufacturers could streamline release testing, propelling the vision of a unified, end-to-end production system even further.
Efficiencies for Increased Demands
The use of nanoparticles and LNPs is expanding beyond traditional applications to include cell and gene therapies (CGTs) for oncology targets. To address the increasing demand for capacity, enhanced processing technologies are essential. New technologies must surpass the limitations of traditional systems, which often struggle with high throughput and yield. Evidence from continuous processing indicates improved yields, reduced waste streams, and decreased cost of goods sold (CoGS) for LNP products. Furthermore, integration of continuous in-line monitoring and control enables CQA assessment and midstream corrections. Continuous processing, along with suitable PATs, offers a solution to overcoming current manufacturing inefficiencies and meeting growing industry demands.
Antonio Costa, PhD, is the chief executive officer (CEO) of DIANT Pharma Inc., and Maik Jornitz is principal consultant at BioProcess Resources LLC; [email protected].
You May Also Like