Take six people and sample the microbial ecosystem on their forearms, and you will find more than 240 distinct microbes (1, 2). So it becomes readily apparent why keeping a cleanroom “clean” is a difficult task. One problem is that we humans are limited in our visual capacities and can see objects down to only 50 µm (3). If we could see contaminating particles, we would likely be very quick to clean them away. In fact, cleaning and cleaning validation are such big issues that they have been cited as the number-one cause of FDA 483s in 2005 (4, 5). But in talking to experts in this area, I found that the key to keeping an environment clean is not in diligent cleaning; rather, it is reducing the sources of contamination.
“One of the biggest problems you have in cleanrooms is people who use gels or facial makeup; women are often the hardest to ‘police’ as they often object to giving up their makeup,” explained Steve Silverman, president of Bartlett Bay Consulting. He clarified that this was not meant to be a sexist comment, just his observation. The more contaminating materials people have on their bodies (e.g., aftershave, hair-gel, lipstick, and rouge), the harder it is to keep the environment clean (not to mention all the microbes already crawling around on their skin). Personal hygiene can cause all sorts of contamination issues, and companies can spend their money actively monitoring all the people that work in the cleanrooms, or they can get the people out of the equation. In fact, independent tests show that the average human generates about 500,000 particles/minute (6).
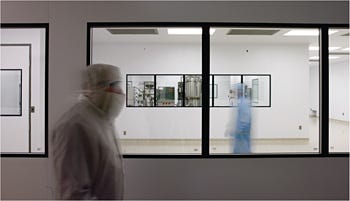
PHOTO PROVIDED BY AES CLEAN TECHNOLOGIES, INC. (WWW.AESCLEAN.COM)
“In the 1980s, the big change in semiconductor manufacturing was between keeping everything clean (people, tools, equipment, product) and keeping the product clean,” explained Silverman. “There are a lot of driving factors, not the least of which are cost and energy, so people said, ‘What we really want is to keep the product clean.’” With cost as a primary driver, the cleanrooms sector moved toward “minienvironments” that kept the product isolated from the operators to minimize the possibilities of contaminants. By now, the design of such spaces has progressed from the early days, and there have been huge benefits in contamination control.
“In the early incarnations of isolators, you found that although theoretically they would be extremely effective in isolating the particular sterile products you’re working on, being able to demonstrate that you had adequately knocked the bioburden down to a level that was suitable for the next production run was a challenge,” says Bikash Chatterjee, president of Pharmatech Associates, Inc. “That’s because there were not enough smooth surfaces — a lot of screws, nooks, crannies, fittings, all those things — opportunities for microbiological intervention. The systems coming out today have incorporated this challenge into their design and are more successful in demonstrating sanitization and sterility.”
Hands-Off Operations
However, new costs arise when you separate operators from products, because products still need to be interacted with in some way: The answer is automation.
“Obviously if you can take the human being out of the equation, the greatest source of your contamination goes away,” explained Chatterjee. “If you can put a robotic system into an isolator, you can get a high level of confidence that your product is sterile.” Although the initial capital investment may be high, the long-term payoff of ensured sterility is worth it.
Air Supply: The most important element in a cleanroom, minienvironment, or isolator is the air. It surrounds everything, and its cleanliness is imperative.
Particle Counters and Air Samplers are the two commonly used cleanroom monitoring tools. Particle counters detect the size and concentration of particles present, and air samplers detect what types of particles are present. Further delineation is made between “viable” and “nonviable” particles, which are used to evaluate the design and control of a CGMP-manufacturing environment (7) (see the “Cleanroom Terminology” box).
The original method for counting particles was visual, through a microscope, and it was a tedious, error-prone process (9). Today, new tools are integrated into facility monitoring systems (FMS) and biological monitoring systems (BMS). Hand-held particle counting units that can collect information at a specific time, but regulatory changes have increased the importance of continuous particle monitoring in cleanrooms (10, 11). An EC guide states that “a continuous measurement system should be used for monitoring the concentration of particles in the grade A zone, and is recommended for the surrounding grade B areas” (10). The FDA states, “We recommend conducting nonviable particle monitoring with a remote counting system. These systems are capable of collecting more comprehensive data and are generally less invasive than portable particle counters” (11). The FDA’s guidance is in line with its process analytical technology (PAT) initiative, providing for continuous quality monitoring and complete process understanding.
Automated systems do not function completely independently. Data are collected using a supervisory control and data acquisition (SCADA) software system and sent to a human monitoring operator located outside the sterile area (5).
Fan Filter Units (FFU): Beyond automated sampling technologies are automated or “smart” FFUs.
“Not only do you have a fan that may be more efficient but it also is an FFU that allows you to control it,” explained Howard Abramowitz, president of AirCare Automation, Inc. “You can control the speed of the fans, you can regulate how much air is moving, and when. Essentially, it’s all built into an FFU.” The interface can be as simple as manual controls to sophisticated stand-alone systems or be tied into building management central control rooms (12).
Not only the control aspects have changed; so have the fans. Abramowitz explained that historically, alternating current (AC) fans were used, but they are not very energy-efficient, produce ambient noise, and have mechanical limitations. The advantage is that they plug in to a standard outlet and are low in cost. New, innovative, smart-AC controls are available that improve AC fan efficiency, reduce mechanical noise, and ease networking control at a low cost.
Electrically commutated (EC) fans come with long-life, low-temperature, “brushless” DC motors that are controlled by maintenance-free electronic circuitry. These fans offer higher energy efficiency and according to Abramowitz are often quieter. The downside is that they require a more intense electrical configuration because they need to have their actual electrical supply converted into brushless direct current (DC) power. This results in higher-cost systems that often exceed the budget limits of cleanroom customers. However, with long-term benefits in energy savings, the higher-cost EC platforms continue to make inroads.
CLEANROOM TERMINOLOGY (6, 8)
Airborne Viable Particulate (AVP) Count: the maximum number of colony-forming units (cfu) per cubic meter of air (or cubic foot of air) that is associated with a cleanroom classification
Air Sampler: equipment used to sample a measured amount of air in a specified time to quantitate the microbiological organisms in a cleanroom
Bioburden: total number of microorganisms detected in or on something
Corrective Actions: actions specified in standard operating procedures (SOP) to be triggered when a problem is encountered
Environmental Monitoring Program: documented policies implemented through SOPs describing in detail the procedures and methods used for monitoring particulates and microorganisms in controlled environments (air, surface, and personnel gear); specified are sampling sites and frequency as well as investigative and corrective actions that should be followed if alert or action levels are exceeded; the methodology used for trend analysis is also described in the SOP.
Nonviable Particle: a particle that does not contain a living microorganism but acts as transportation for viable particles.
Out-of-Specification (OOS) Event: a temporary or continuous occurrence wherein one or more requirements in the SOPs for controlled environments are not fulfilled
Sampling Plan: a documented plan describing the procedures and methods for sampling a controlled environment; identifies sampling sites and frequency and the required number of samples; describes the method of analysis and how to interpret its results
Sampling Sites: documented location within a controlled environment where samples for microbiological evaluation are taken
Sterility: in the strictest sense, a complete absence of viable microorganisms
Viable Particle: a particle that contains one or more living microorganisms
“The industry has moved from centralized air blower systems with vents to fan filter units, and those people at the very high end of the spectrum have been willing to pay extra for fan filter units that have the more expensive fans in them because it gave them control,” said Abramowitz. “The fact that it saved energy was an added benefit that helped offset some of the extra costs.”
Historically, the system of choice for controlling these fans is a customized PC-based program configured and customized for each installation — also known as “central control” platforms (12). As can be imagined, such systems require a high initial investment that makes them too costly for some applications. Now on the market are preprogrammed logic controller (PLC) or dedicated consoles that implement control of a single “zone” of smart FFUs (12). Such systems are available off-the-shelf with predesignated features and functions that can be deployed with little to no software modification. They can use a stand-alone interface controller or be integrated into a central control platform. Beyond offering control, the units also save space and operator time for adjustment.
“With a central blower you need a lot of duct-work and all kinds of dampers and controls to control how much air went to each filter,” said Abramowitz. “All of that took up a lot of room, and it took up a lot of time and effort to get the dampers and everything set. It also was taking up a lot of ceiling space.” He explained that it was a cumbersome process to make adjustments, it took a long time, and it was expensive work. The fan filter units are smaller self-contained units that can be easily adjusted independently. And at nearly two feet by four feet, they don’t take up much room in a ceiling.
The FFUs are also helpful with the minienvironment concept. With smaller fan units that can control airflow in smaller spaces, the one-size-fits-all approach of historic systems is no longer a problem. Additionally, because cleanrooms don’t always need to be pressurized at the same level or circulating air at the same rate (for example, when not in use) smart FFU systems can save companies a significant amount in energy costs.
“We’ve seen in rooms where you tailor the performance of the FFUs that you can save 30–40% of the energy costs right off the bat, just by allowing the system to be optimized or to be adjusted to what’s really needed as opposed to just running full out,” shared Abramowitz. In fact, in an example of a retrofitted AC FFU system, cost savings on energy alone came in at $5,500/year (13).
Air Flow Modeling: “In the end, everything comes down to designing the air flow within the environment,” explained Silverman. “If you have a lot of air turbulence, all bets are off. You could have the best filter in the world, and you could have a very dirty air flow.” Using computational fluid dynamic (CFD) modeling, you can input the parameters of your cleanroom or minienvironment and model the airflow with a visual representation. CFD uses sophisticated software programs that use numerical calculations to solve the complex partial differential equations to describe turbulent flow (14). Vendors such as Fluent (owned by Ansys, Lebanon, NH, www.fluent.com) and Flomerics (Surrey, United Kingdom, www.flomerics.com) license their software to companies, which can then model the airflow in their cleanrooms. CFD modeling helps confirm phenomena that result from “good-sense” physics that are impossible to measure or hard to visualize (14).
“CFD is like any other tool available,” said Silverman. “Success doesn’t depend solely upon the tool itself; it depends greatly upon the person that’s doing the modeling. I once was in charge of a CFD project for a company, and it took me a couple of years to really understand and fully use it.”
Single-Use Systems: Beyond air circulation and filtration technologies, another boon to cleanrooms are single-use processing systems. These presterilized systems have become prevalent in the biopharmaceutical industry. Their advantages include (15)
Eliminating cleaning and cleaning validation
Eliminating sterilization
Reducing use of caustic chemicals and detergents, as well as water for injection (WFI) consumption
Allowing efficient use of space, with smaller footprints and reduced storage requirements
Reducing cross contamination
Reducing downtime associated with stainless steel assembly, labor, CIP, and SIP
Providing the ability and flexibility to increase production capacity.
Single-use systems are sterilized by the application of electromagnetic radiation (gamma rays) emitted from radionuclides such as Cobalt 60 (60 Co) and Cesium 137 (137 Cs) isotopes (16). With gamma irradiation, microorganisms are inactivated by damage to their nucleic acids. When implemented, these sterile systems can be used for media and buffer preparation, fermentation, fluid transfer, filtration, and fill and finish operations. Their presterilized nature allows them to be more of a “hands-off” system because they remove the need for manual cleaning and related cleaning validation operations associated with that type of set-up.
Single-use systems have further advanced in the speed of connecting new components in an aseptic environment. The Pall Kleenpak connector can make a connection anywhere in a facility in seconds because it does not require the use of a laminar flow environment, or any other capital equipment (17). More time-consuming options include the use of “quick connections” or tube welders under laminar flow hoods.
Some concerns about the contamination potential of single-use systems in processing systems are addressed in the “Communication, Education, and Initiative in Bioprocessing and Biopharmaceuticals” box.
Fill Operations: The last step in producing a biopharmaceutical product is the filling of vials, ampules, and syringes. This step must be done in an aseptic environment to prevent final-product contamination. Technological innovations have enabled aseptic filling facilities to more easily ensure a high level of contamination control.
“We’re also seeing single-use aseptic filling systems that are incredible,” said Chatterjee. “They can use a variety of different fill configurations, and they use a variety of single-use tubing and fill heads. It’s really a fabulous way when you integrate it with one of the isolator technologies to drive your sources of contamination down to almost nothing.” These systems can be automated with robotic systems, increasing the sterility of the actual filling operation.
An example of such integrated single-use filling systems was recently described in an article from Vibalogics (18). The company integrated a single-use filling head, the Acerta DS1 dispensing system (Millipore Corporation, Billerica, MA, www.millipore.com). Seamless integration of the dispensing system into existing filling lines was ensured by a basic “handshaking” protocol: The dispensing system dispenses only when specific conditions are met; then a signal is given to the filling line to move the vials forward. The dispensing system successfully dispensed volumes within 0.2 mL and 10 mL, with accuracy of <0.5% in weight (19).
Training Is Key
Regardless of a move to get people out of the equation, operators are still necessary to cleanrooms. From clean-up to monitoring and process equipment maintenance, operators at times will be required to be in the cleanroom environment. Because human-interaction is the largest source of contamination, it is therefore imperative that people are well trained in cleanroom protocols.
“Very often the weakest link in cleanroom contamination control is the gowning procedure, where the gowns are kept, and the maintenance of the gowning room, which is often overlooked,” explained Silverman. “People are trained, but very often they’re not monitored closely, and the whole process becomes compromised quite easily.”
Chatterjee explained that the keys to a good cleanroom training program are basic job-skill training, having an apprentice program, and combining that with book-learning to understand the weaknesses of the processes, the steps that you’re going to follow to ensure the product safety, and the dos and don’ts of the cleanroom. For more advanced applications such as stem cells, monoclonal antibodies, or gene therapies, Chatterjee explained that he has found more and more cleanroom operators to be highly skilled, possessing a Masters degree or even PhD in microbiology. “The challenge is that when you get more sophisticated in your contamination control architecture, the skill-set of operators in that area goes way up,” said Chatterjee.
COMMUNICATION, EDUCATION, AND INITIATIVE IN BIOPROCESSING AND BIOPHARMACEUTICALS
Moving from CGMP to future GMP is a major challenge for biopharmaceuticals. Meeting this challenge requires communication, education, and initiative. Although plastics fabrication and metal processing systems are inherently less complex than biological systems, such products are subject to greater variability than might be expected. Any change in the components involved in a manufacturing process is a potential problem. Additionally, defining what constitutes a change requires careful wording of documentation and clear communication with suppliers.
Given the competition-sensitive nature of the formulation and processing of plastics components, an end-user’s knowledge of the process may be incomplete. Therefore, it may be necessary to qualify acceptable bioprocessing components and to obtain agreement from a supplier to “freeze” the process and inform you when a change is contemplated. This concern is not limited to polymers; the attributes of metal components can also change. Lubricants, cleaning agents, and manufacturing processes associated with their use are subject to modification. Such fluids are often complex blends, and formulation changes may be beyond the control of the supplier. For example, high-boiling, biobased cleaning agents may be adopted because of their preferred lower environmental impact, but they can leave significant residue.
A second major challenge is the potential negative impact of batch-to-batch variability on bioprocessing. For example, when packaging and processing systems are validated, the levels of leachable and extractable contaminants may be found acceptable for a given batch of plastics. When new batches of components are used, the potential for significant process change is significant. Identifying, tracking, and monitoring the batch-to-batch variability of each processing or packaging component is critical. So is communication with each supplier of components.
Third, we need to add the concept of initiative to that of “dos and don’ts.” Typically, when our clients ask us for help with contamination control and critical cleaning processes, what is envisioned is a long list of “dos” and “don’ts” that everyone from engineers to lab technicians must follow. Standards, lists, procedures, controls, and automation are essential in bioprocessing, whether they specify how to properly don protective clothing or when to check for variability in plastic tubing. However, if simple standards and lists were sufficient, most manufacturing processes could be conducted by robots. The advantage to human operators is that they can go beyond a robotic skill-set. Humans can notice when conditions change and make complex judgments based on those observations. Employees cannot be preprogrammed for every exigency, but they must understand not only the “how” of the process but also the “why.” Leaning “how” is training; knowing “why” is education.
A well-educated employee can save a process. This is especially important with complex biological systems. For example, in a recent critical application we know of, a processing solvent that should be colorless was received with acceptable vendor certifications. However, an observant employee noticed that the solvent had a pink coloration and, understanding that was not the expected appearance, immediately questioned whether the material should be used. Many acceptance protocols and automated fluid handling processes would not have been programmed to detect and act on the anomaly.
Having educated and communicative suppliers is also critical. When a vendor employee understands not only the physical and chemical specifications but also the eventual use of a component, he or she can be a true partner in providing the best value for your product.
Barbara and Ed Kanegsberg, BFK Solutions LLC, are independent consultants with decades of experience in critical cleaning, assembly, and contamination control. Their projects span life science related processes to complex, high-volume manufacturing. 1-310-459-3614 or .
The people taking the training are changing as well. Consultants Barbara and Ed Kanegsberg (www.bfksolutions.com) shared that the number of suppliers and subvendors are attending their workshops. “We see increasing interest on the part of the suppliers and subvendors in what the ultimate use is of the product they’re providing, and how they can provide better reliability for process control and contamination minimization,” explained Ed. Barbara noted that this was a change from the past when efforts to resolve contamination-related issues would be met with an attitude of “Well, we have our standards, but the quality assurance and minimizing leachables and extractables is the responsibility of the customer. They’re realizing that the complexity of contamination control in biopharmaceutical and biological products require their active participation, their active understanding, and their active education.”
One of the biggest problems noted by all the consultants I talked with was the issue of complacency within companies about GMP and aseptic cleaning. Silverman expressed it best: “You know the saying that the road to hell is paved with good intentions? Well in my experience, companies often start out very good and they’ll hire someone to come in and give training sessions,” said Silverman. But with turnover and time, Silverman fears that after two or three years there will be a whole new crop of people who instead of learning from an expert, got only the “apprentice” program from an operating technician who maybe wasn’t able to explain the importance of the order of those steps. So the new people are missing some of the elements of fundamental understanding that are important to a good contamination control program. And being the cleanroom monitor is not exactly the most prime job.
“To have a cleanroom trainer or somebody monitoring is not something people like to do because they’re not particularly rewarded for it,” explained Silverman. “Companies reward people for good ideas but not for going to a cleanroom and reporting that somebody’s wearing eye shadow.” Silverman continued that every company knows this is a problem, and every company struggles with it. “Basically, it’s not a fun or rewarding job to be the cleanroom police.”
The Future: Cleaner and Cheaper
Leading the way in cleanroom technologies and improvements is the semiconductor industry. With ISO Level 1 minienvironments, semiconductor companies have integrated a high-level of automation into all aspects of their processing, and they have embraced minienvironments to isolate product from operators to ensure a high degree of sterility in manufacturing.
“Automation technology has been improving,” said Silverman. “What has really, really pushed the envelope in terms of the cleanliness is this overall computer modeling and improved design. That has gotten us down to a level that right now we’re approaching some of the limits in cleanliness. We just can’t get it any cleaner.” Silverman explained that over the past 10 years the cleanrooms and minienvironment sector have been seeing up to ∼20% improvements per year. The ISO levels outlined in ISO 14644 are based on the number of particles of a certain size (in µm) per cubic meter of air (19). Those standards superseded the Federal Standard 209E: Airborne Particulate Cleanliness Classes in Cleanrooms and Clean Zones on 29 November 2001. ISO level 1 is the cleanest level, allowing only 10 0.1-µm particles and two 0.2-µm particles per cubic meter (0.283 p/ft3 and 0.057p/ft3, respectively).
Using existing and yet-to-be-developed advanced technologies, the biopharmaceutical industry is following on the heels of the semiconductor industry, moving from ISO 5, 6, and 7 down to levels 2 or 3. This can be done through the use of restricted access barriers (RABs) or isolator technologies.
Additionally, thinking about what really needs to be clean and getting the rest of the operation out of the cleanroom is cost-effective and is essential to achieve the required quality. “There’s a tendency on the part of manufacturers in general to squish as much of the process into the cleanroom as possible,” said Barbara Kanegsberg. “They are assuming that the process will be clean — kind of like the old Maxwell Smart, everybody squishing into the cone of silence to make everything safe. If you have a process that degenerates particles, thin-film contamination, or some other kind of dirt, you have to control that process, and maybe you don’t want to put it in a cleanroom.” Moving away from having everything inside a cleanroom can be more cost-effective, because noncleanroom areas do not require the same level of intense air flow control or particle monitoring and validation.
There will surely be further improvements in the air systems of the future. Already Silverman is seeing suppliers developing filters with electrostatic charges to remove more particles from the air. Perhaps nanotechnologies will add additional contaminant-removal capabilities to air systems.
“Some new things are happening in filters,” said Silverman. “Rather than being passive, they’re going to be active — for example, putting a charge on the filter materials to make it an electrostatic filter. They’re starting to talk about that to get ultraclean air.” Silverman explained that currently, the best filter on the market that he’s aware of can capture down to ∼ 0.12-µm particles. But Silverman predicts that filtration systems will soon be looking at capturing much smaller particles using improved filter media.
The future looks bright and squeaky clean.
REFERENCES
1.) Gao, Z, C Tseng, Z Pei, and MJ. Blaser. 2007. Molecular Analysis of Human Forearm Superficial Skin Bacterial Biota. Proc. Natl. Acad. Sci. U.S.A. 104:2927.
2.) Harder, B.. Humans Wear Diverse “Wardrobe” of Skin Microbes, Study Finds.
3.) Seigerman, H. 2007.Point of View: The Problem Is, We Can’t See the Problem — Or……Our Eyes Deceive Us Controlled Environments Magazine.
4.) McCormick, D. 2005. FDA’s Evans Reviews Causes of Warnings and Recalls. Pharm. Technol. http://pharmtech.findpharma.com/pharmtech/content/printContentPopup.jsp?id=190230.
5.) McCormick, D. 2006. Poor OOS Review Leads Causes of FDA Citations. Pharm. Technol. http://pharmtech.findpharma.com/pharmtech/content/printContentPopup.jsp?id=383878.
6.) Kelly, J. 2006. Particle Monitoring: The Bugs You Can’t See. Controlled Environments Magazine www.cemag.us/articles.asp?pid=618.
7.) Mackler, SE. 2004. Environmental Monitoring: Particle Counts Are Easy. BioProcess Intl. 2:54-61.
8.) MacAteer, F, and J. Barbato. 2004. A Primer on Viable Particles Monitoring. BioProcess Intl. 2:56-57.
9.) Babb, J. 2007.Understanding Particle Counting Technology CleanRooms.
10.) European Commission 2003. EC Guide to Good Manufacturing Practice Revision to Annex 1: Manufacture of Sterile Medicinal Products.
11.) 2004.United States Food and Drug Administration, Center for Biologics Evaluation and Research Guidance for Industry: Sterile Drug Products Produced by Aseptic Processing-Current Good Manufacturing Practice.
12.) Abramowitz, H. 2007.Reducing Cost and Complexity of Managed Cleanrooms Controlled Environments Magazine.
13.) Fishbein, J. 2006.AC Fan-Driven Cleanroom Fan Filter Units Benefit from TRIAC and Network Control CleanRooms.
14.) Brochet, A, and A. Solberg. 2002.Enhancing Cleanroom Design with Airflow Modeling CleanRooms.
15.) Cardona, M, and B. Allen. 2006. Incorporating Single-Use Systems in Biopharmaceutical Manufacturing. BioProcess Intl. 4:10-14.
16.) The Irradiation and Sterilization Subcommittee of the Bio-Process Systems Alliance 2007. Guide to Irradiation and Sterilization Validation of Single-Use Bioprocess Systems, Part 1. BioProcess Intl. 5:32-39.
17.) Haughney, H, and H. AranhaSingle-Use Products Dispose of Cleaning Cleanroomsfaq.blogspot.com.
18.) Paginini, G, and L. Machulez-Hellberg. 2007. Single-Use Technologies Bring Flexibility to Final Filling Operations: Successful Integration of a Disposable Liquid Filling System. BioProcess Intl. 5:84-87.
19.) 2001.Institute of Environmental Sciences and Technology Offical ISO 14644 Standards.