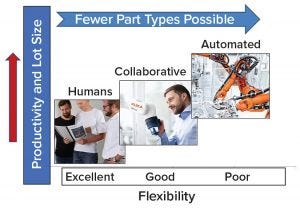
Figure 1: General principles for application of collaborative robots in manufacturing
It should come as no surprise to anyone familiar with biomanufacturing that current designs of bioprocess facilities as well as associated manufacturing spaces and support operations require excessive amounts of manual labor and manual interventions that lead to high labor costs and, consequently, total cost to supply. From receipt of raw materials to process execution and performance review, resolution of quality issues, and product shipping, no industry devotes a greater percentage of operating costs (or cost of goods sold, CoGS) to labor than biomanufacturing. For example, 35–50% of labor cost is typical in biomanufacturing (1, 17) compared with 10–14% for the next highest industry (1, 2). Increasing availability of in-line sensor technologies, adoption of paperless manufacturing and laboratory execution systems (MES/LES), integration of multiple control systems, automated processing without manual intervention, and targeted application of mobile robotics are expected to decrease substantially the required labor and associated costs of biomanufacturing.
Increasingly, robots are executing many routine operations (e.g., material handling, waste handling, material transport, and hazardous operations), autonomous operations, and challenging tasks such as precision machining (3). In parallel, robots are being developed to be smarter, less expensive, and more versatile than ever before. Many have been designed specifically to operate safely in close proximity to human workers (e.g., collaborative robots or cobots) and increasingly have been implemented by many industries (3, 30).
Robotic systems first started making inroads in the biopharmaceutical industry through the implementation of high-throughput automated systems for drug discovery and analytical assays. Robotic systems now are making inroads in process development with the introduction of ambr (Sartorius Stedim Biotech GmbH) and Tecan (Tecan Group Ltd.) systems. In the next few years, our industry is likely to see more applications of robotic systems, particularly mobile and collaborative robots in warehouse and production floors of biomanufacturing facilities.
Here we introduce and create awareness of potential applications for robotics in biomanufacturing, availability of robotic systems, and incentives for robotics suppliers to adapt existing systems or develop new solutions for the biomanufacturing industry. And we highlight key challenges in implementing mobile robotics in biomanufacturing. To our knowledge, this is one of the first publications covering robotic applications for the biomanufacturing industry. A team of industry experts coordinated by the BioPhorum Operations Group (BioPhorum, London, UK) assisted with critical discussions and data gathering for this introductory article.
Scope
Until a few years ago, traditional biomanufacturing facilities consisted of two- to six-pack, large-scale (>5,000 L) stainless steel (SS) bioreactors and ≥1-m diameter chromatography columns. With the advent of single-use (SU) production systems, higher product titers and recovery, and lower annual demand, the longer-term trend is toward construction of SU facilities operating at an intermediate scale of ≤4,000 L with open floorplans (4, 5). In parallel, increasingly diverse products are under development. They include whole cells (cell therapy) or viral vectors (gene therapy), which challenge traditional biomanufacturing facility designs with respect to manufacturing scale and segregation.
However, given that the industry remains heavily reliant on recombinant protein manufacturing (SS large-scale and SU medium-scale facilities), the scope of this article is limited to recombinant protein drug substance manufacturing and excludes research and development (R&D) and quality control (QC) laboratory applications in which multiple fixed robotic systems are already in use (6). It also excludes fill and finish operations where significant automation is already in place (7) as well as cell and gene therapy manufacturing.
Robotics and Manufacturing
Robotics is expanding from automotive and electronics into new industries and applications, including the biopharmaceutical industry. Smaller, lighter robots (compared with those at industrial scales) that are safe to operate in close proximity with humans (cobots) (Figure 1) are being implemented increasingly. Traditionally, robots in manufacturing perform dull (repetitive), dirty, or dangerous tasks (3). The number of dirty or dangerous tasks in biomanufacturing is limited (e.g., depopulating filter housings or lifting heavy objects such as media containers). However, unlike high-volume manufacturing (e.g., “making widgets”), biomanufacturing has a comparatively lower frequency of repetitive tasks and higher diversity of operations. Modern biomanufacturing facilities consisting of multiple bioreactors and production trains are likely to be more suited for cobot applications following the illustration in Figure 1.
Biomanufacturing involves different manual operations — from material receipt in warehouses to sampling and multiple preparation activities. Adding to the documentation burden, most operations require extensive chronological documentation, a record of the employees who performed the operations, and (for certain critical applications) a second verification. Most manual operations in biomanufacturing involve raw materials or consumables, samples, and preparation activities.
Senior management personnel at several biologics manufacturers were surveyed to understand key drivers for implementing robotics (e.g., reducing human effort, increasing ergonomics and safety, and reducing documentation). If a particular suboperation involved more effort on documentation than physical labor, then it is more amenable for an electronic documentation solution rather than a robotic solution.
For this survey, the three key operation categories (raw materials or consumables, samples, and preparation activities) were subdivided further into 16 suboperations, and respondents were queried on the drivers for reducing operating costs. Respondents were biomanufacturing site leaders from different companies operating primarily fed-batch processes in multiproduct facilities containing multiple product trains with 2,000 L to 20,000-L production bioreactor scales.
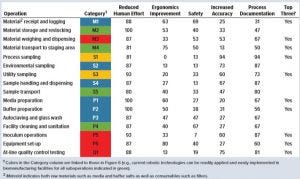
Table 1: Percentage of survey respondents indicating key drivers for reducing operating costs in biomanufacturing operations
Table 1 shows the percentage of responses in each category. The overwhelming majority of responses indicated that reducing human effort required for all 16 suboperations would reduce operating costs significantly. Several respondents also indicated that reducing the documentation effort would benefit a few suboperations. Respondents also were asked to name the top three suboperations that should be targeted for reducing the human effort required through application of robotic technologies. In that case, responses were mixed, but several respondents indicated a few operations pertaining to materials, samples, inoculum laboratory operations, and at-line QC testing. These results confirm that significant human effort is expended in biomanufacturing. Several operations executed daily should be examined in depth for application of robotic technologies.
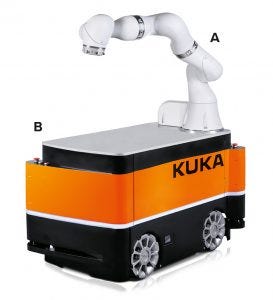
Figure 2: A 7-Axis Cobot LBR iiwa (intelligent industrial work assistant) from KUKA (A) mounted on a KUKA mobile platform KMP 200 (B) (IMAGE COURTESY OF BRIAN COONEY, KUKA ROBOTICS)
Modern Robotics and Applications in Biomanufacturing
Some companies (e.g., Kuka, ABB, Fanuc, Universal Robots) offer cobots with diverse features (e.g., payload, reach, weight, and price) for safe operation alongside human operators (8). Figure 2 shows one example. Such lightweight cobots were introduced relatively recently (<10 years ago). They are being used more and more in manufacturing operations to perform both simple pick-and-place tasks and more complex assembly tasks. We can imagine such cobots with suitable modifications operating in warehouses or on shop floors assisting operators with receiving materials, process samples, and glass washing. The biomanufacturing industry could learn well from Amazon’s experience: As of May 2017, Amazon is using over 80,000 robots in its warehouses globally with plans to add more (8).
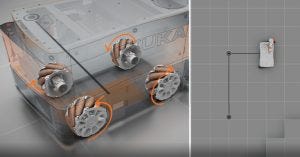
Figure 3: Mobile robot with omnidirectional wheels, which enables backward, forward, sideways, and rotational motions
At the same time, several notable advances have been made in mobile robotics. Autonomous mobile robots (AMRs) can move not only forward and backward (Figure 3), but also sideways (a great feature for legacy facilities that have limitations of free movement). They can slow down or stop when a human operator is present as well as call and ride elevators (9). They represent a significant improvement over automated guidance vehicles (AGVs), which can move only on predesignated paths. Industrial-grade autonomous robots can find their way around to an accuracy of 1 mm, have an arm with the accuracy of 0.1 mm, and are safe to operate next to human beings. Not many people know this technology exists, and even fewer have realized its potential.
Certain applications such as transporting materials from a receiving dock to a storage location in a warehouse and from that warehouse to a staging area or material airlocks (MAL) can be performed easily by commercially available mobile robots. Mobile robots also can be used in cleanrooms for transporting buffer bags from buffer preparation areas to purification suites. Indeed this application is being pursued in one author’s former facility. An excellent conceptual demonstration of mobile robots delivering media and buffer totes is shown in the GE BioPark biomanufacturing campus virtual tour (16).
A good example of a purpose-built application of a mobile robot is the Neo line of autonomous cleaning robots developed by Avid Bots (11). Neo robots seamlessly integrate state-of-the art sensor technology using lasers and three-dimensional (3D) cameras, with artificial intelligence (AI) navigation and innovative product design (11). Neo robots were designed with safety as a priority. Industry-leading safety features include collision avoidance technology, protective bumpers and flaps, an e-stop button, warning lights, and a low 72 dBA noise rating (11). The current generation of such robots can be implemented easily for cleaning floors in warehouses and cleanrooms in biomanufacturing facilities.
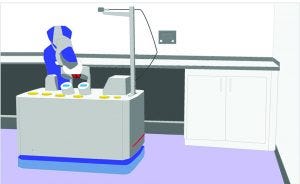
Figure 4: Conceptual design of a mobile collaborative robot for environmental sampling
Given the descriptions above, it’s not surprising that the current trend is to mount a cobot on top of an AMR, thus increasing the cobot’s versatility and applicability (10) (Figure 2). Indeed, use of mobile collaborative robots in warehouses has increased enormously, and the current trend is toward automation of every operation in logistics (12). Such robots can be used easily in warehouses for storage and retrieval of raw materials and consumables. Figure 4 shows another possible application in environmental sampling in cleanrooms.
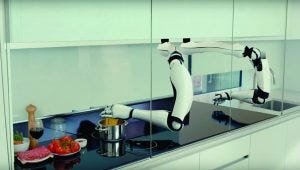
Figure 5: Moley Robotics automated kitchen (REPRODUCED WITH PERMISSION FROM MOLEY ROBOTICS AND INVENTOR MARK OLEYNIK)
PM Group has assembled a consortium consisting of end users and members of academic/research institutes to conduct a feasibility study on the use of AMR for the above application. It is our intention to share results of this project in a future publication.
An internet search for the term dispensing robots yields many hits, and many automated liquid and solid-powder dispensing robots are available (13). Currently, most of these robots operate at small scales (≤100 mL of liquid or ≤100 g of powder). It is conceivable that future improvements would lead to robots that can dispense much larger quantities of liquids or solids. Another feature seen increasingly is the capability for combining different unit operations such as dispensing and mixing. Indeed, the robotic kitchen concept (Figure 5) can be implemented for automating preparation of small volumes of media or buffers (14). Such robots could operate in biomanufacturing plants in collaborative or autonomous ways. Future generations can be scaled up to produce larger quantities of media or buffers. Although requirements of biopharmaceutical production are different from those of a kitchen, they are similar with respect to flexibility and complexity of the handling process.
Yet another example of specialty robots is of those designed to perform minimally invasive surgeries (15). These systems have multiple arms required for simultaneously performing different operations unlike the cobots described above (15). The imaginative mind can now think of using such multiarm robots for bioprocess equipment set up, for example.
The applications described above involve primarily human-directed robot operation. It is conceivable that future facilities will integrate robot operation with automation systems such as plantwide control systems (PCS), MES/LES, and enterprise resource planning (ERP) systems. Thus, a particular raw material reaching critical stocking level in media preparation, for example, could trigger automatically through a company’s ERP system a request for restocking to an AMR. Such a scenario is an impetus behind Industry 4.0 operation and is explored further in another section below.
This section has overviewed the types and variety of robots available. We encourage readers to review the images and videos provided in the hyperlinks in the reference section to fully appreciate the advances in modern robotics and determine possible applications in biomanufacturing. The overview is by no means a comprehensive review of available robots. Additionally, a multitude of accessories such as grippers, cameras, image processing software (together with the robot) can be adapted or designed from scratch for application in biomanufacturing. Currently available cobots, mobile collaborative robots, or specialty robots are offered primarily for the general manufacturing or consumer industry, and to our knowledge, no known entities are developing cobots specifically for biomanufacturing.
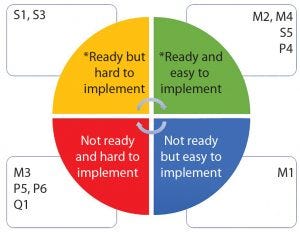
Figure 6: Biomanufacturing suboperations classified by technology readiness level and ease of implementation (*ready = technology available, categories defined in Table 1)
Armed with at least a basic knowledge of modern robotics and deeper knowledge of biomanufacturing, we classified the tasks shown in Table 1 into four categories based on availability of robotic technology and ease of implementation (Figure 6). It’s not surprising that operations involving transport of materials or samples are easy to implement using available modern robots. By contrast, operations involving multiple subtasks (e.g., equipment set-up and inoculum operations or high-accuracy dispensing) are more difficult to implement because suitable robots are unavailable, and there is a need to conform to good manufacturing practice (GMP).
Challenges in Implementing Robotics in Biomanufacturing
The previous sections emphasized a strong case for reducing the amount of human labor expended during biomanufacturing and the potential for modern robotics to help manufacturers achieve that goal. A key factor behind the slow adoption of these systems is that biomanufacturing industry personnel generally do not know about advances in robotics. Low interest from robot suppliers in developing new products for biomanufacturing stems from a higher economic attractiveness and easier product development for general manufacturing and the consumer products industries. Below, we focus on key challenges in implementation of robotics in biomanufacturing.
Facility and Equipment Layout: Biomanufacturing facilities typically are multilevel and can have ladders, elevators, and dumb waiters for transporting personnel, materials, and samples. As mentioned above, AMRs can call and ride safely in elevators and dumb waiters with operator assistance. However, they cannot use ladders, so they have certain limitations on their free movement. Legacy facilities can pose an additional challenge where the equipment layout is not ideal for free movement. Thus, an AMR with omnidirectional wheels that permit forward, backward, and sideways motions is ideal (Figure 3).
Another challenge posed by legacy biomanufacturing facilities is movement between classified areas such as from Class D to Class C environments and vice versa. However, recently introduced misting tunnels might provide an ideal solution to simplify material transfer between nonclassified and classified areas (18–21). Indeed, it is tempting to think that future facilities may have the material airlocks for automatically spraying and wiping down not only small parts but also large containers and robots. Touchless car washes for fully automated cleaning of cars and small trucks already exist, and one can imagine that the same technology may be adapted in biomanufacturing facilities.
Finally, future facilities with an open ballroom concept design would be more conducive to free operation of mobile robots, and they should be purposefully designed to incorporate modern robotics in operation. It is also worth mentioning that PM Group is working with Technological University Dublin to facilitate movement of robots between different grades of classified areas.
Despite the above challenges, for certain cases such as transporting materials from warehouse to material airlocks, robotics can be implemented easily because the technology is sufficiently advanced, and the robot movement occurs primarily outside of cleanroom environments, with no movement across different room classes.
Nature of Tasks and Equipment Compatibility: It goes without saying that certain simple tasks such as pick-and-place operations involving parts of identical shape are easy to automate. Considerable advances have been made in gripper technology and the advent of soft robotics grippers (22). Such innovations promise to simplify tasks involving the picking up of parts of different shapes, sizes, and weights using a robot — a feature that will help immensely with material receipt and storage in warehouses.
Tasks requiring at least two arms (e.g., making a tri-clamp connection) are more difficult for existing robots. When faced with these challenges, manufacturers have resorted either to using more robot-friendly equipment design (e.g., bioreactors shaped like Tic-Tac boxes developed by Tap Biosystems for its ambr system) or adapting the robot (e.g., Andrews Alliance robot, which works with commonly used pipettors) (23). Revisiting the EM monitoring project described above, existing designs of settling plates and touch plates are robot friendly, whereas viable and nonviable monitoring equipment designs are not.
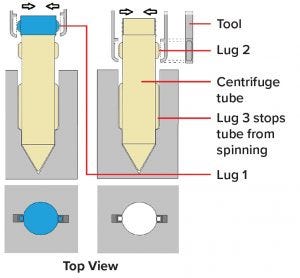
Figure 7: Design of robot and human compatible centrifuge tube (PM Group patent pending and Irish Application No S2018/0573)
A middle ground is equipment design that is both human operator and robot friendly. One example would be to change the design of a centrifuge tube so that a robot can use light force to remove the lid and make pick-and-place operations reliably without compromising human ergonomics (Figure 7).
Competing Technology, Cost of Development, and Return on Investment (RoI): As with any other new technology, robotics will have to compete with both existing technology and competing technology. For example, mobile autonomous robots can be adapted for sampling purified water or water-for-injection (WFI) loops for biochemical and microbiological analysis. However, recently introduced systems can perform real-time, on-line, and off-line instantaneous microbial detection in water systems (23, 24). Another competing technology can sample the environment for both total particle count and viable particle monitoring (25). It will be interesting to see which technology wins out over the longer term. It might be worth exploring whether mounting an instantaneous air detection system on a mobile autonomous cobot might solve both the issues of human labor expended in environmental sampling and the incompatibility of current sampling devices with robotic technology.
Introducing new technology to any biologics manufacturing facility is a complex process and places undue burdens on biomanufacturers to demonstrate that the new technology is providing comparable results with comparable (or better) accuracy, reproducibility, and variability (26). In addition, the capital and resources expended in evaluating and implementing such technologies should be recovered in a timely manner. For these reasons, the initial application of modern robotics is likely to be in operations not subjected to GMP compliance, such as transporting materials or samples. However, once experience with robotic systems is gained, they could be allowed to perform applications subjected to GMP such as environmental sampling.
Technology development involves taking some risks, incurring significant costs, and expecting a timely return on investment. Robot developers understandably are concerned about developing products for an industry that they poorly understand, especially one with a significant regulatory burden for introducing new technologies. Continued dialogue, exchange of needs, and communication of advances in technology between biomanufacturers and robotic suppliers will be keys to realizing the benefits of modern robotics for biomanufacturing. The authors hope that this publication provides the necessary impetus for fostering such a dialogue.
Modern Robotics and Smart Manufacturing and Industry 4.0
A recent trend in manufacturing is to implement increased automation and data exchange, thus leading to a smarter manufacturing approach. Such efforts are combined with a gradual progress toward realizing the benefits of the fourth industrial revolution (Industry 4.0) and its interconnected cyber physical systems essentially operating without human intervention. Modern robotics combined with other smart industry enablers such as artificial intelligence (AI), data analytics, mobile devices, scale-out, systems integration, and digitalization will help biomanufacturers overcome multiple roadblocks. Progress in companion technologies (sensors, end-effectors, adaptive and reconfigurable systems, intuitive programming, systems interoperability, and cooperative and collaborative approaches) and increasing affordability also will trail-blaze the path for robots into small and midsized biomanufacturing facilities.
Although the scope of this discussion was limited to traditional recombinant protein manufacturing, smaller, more modular-sized facilities for cell and gene therapies and individual product manufacturing such as chimeric antigen receptor T cells (CAR-T cells) and autologous cell therapies also will benefit by the implementation of robotics. Taken together, robotics and other Industry 4.0 enablers will play a key role in transforming the entire biomanufacturing industry.
The experience from other industries that have implemented robotics is positive. In the automotive industry, studies have shown that teams made of both humans and robots collaborating efficiently can be more productive than teams made of either humans or robots alone, and the cooperative process reduced human idle time by 85% (27). The International Federation of Robotics has concluded that the introduction of robots increases productivity and competitiveness leading to increased demand, without drastic negative effects on employment levels (28). The Wall Street Journal also stated that the introduction of small collaborative robots has the potential to make American manufacturing competitive again (29). Thus, the time is ripe for the biomanufacturing industry to strongly consider robotics in its daily operations.
Discussion
We have presented a case for reducing manual labor — a key challenge in biomanufacturing — by smart and targeted application of modern robotics. Biomanufacturing subject matter experts (SMEs) have confirmed that significant human labor is expended during multiple operations performed routinely during biomanufacturing. We presented several examples from other industries about the versatility of modern robotics to serve as catalysts for applications in biomanufacturing. We believe that the biomanufacturing industry is ready for implementation of modern robotics. As with any new technology, several challenges exist. Awareness of the challenges and opportunities is important to navigate the tricky path from conception to implementation.
We hope that our discussion will kindle interest in both the biomanufacturing and robotics communities simultaneously. What is required now are intrepid operators and engineers supported by visionary management with an appetite for risk-taking and a shared goal of bringing about much-needed efficiency gains in biomanufacturing facilities.
Acknowledgments
We acknowledge the assistance provided by the BioPhorum Operations Group (BPOG) personnel Robin Payne and Graeme Moody in facilitating team meetings, providing encouragement, and fostering open communication. Graeme in particular was instrumental in designing and compiling the survey. The BPOG robotics team members, David Lehmann (MilliporeSigma Merck KGaA), Thorsten Kimmel (Roche), and Christoph Lebl (Lonza AG) provided timely review and critical comments and new ideas. Finally, special thanks to Brian Cooney of Kuka Robotics for providing high-quality images.
References
1 Sinclair A. How Geography Affects the Cost of Biomanufacturing. BioProcess Int. 8(3) 2010: S16–S19.
2 Cost of Labor, Cost of Goods Sold. BizStats; www.bizstats.com/reports/cost-of-labor.php.
3 The New Hire: How a New Generation of Robots Is Transforming Manufacturing. PricewaterhouseCoopers LLP: New York, NY, 2014; www.pwc.com/us/en/industrial-products/assets/industrial-robot-trends-in-manufacturing-report.pdf.
4 Seymour P, Ecker D. Global Biomanufacturing Trends, Capacity, and Technology Drivers: Industry Biomanufacturing Capacity Overview. Amer. Pharm. Rev. 30 June 2017; www.americanpharmaceuticalreview.com/Featured-Articles/188840-Global-Biomanufacturing-Trends-Capacity-and-Technology-Drivers-Industry-Biomanufacturing-Capacity-Overview/.
5 Jones S. BioPhorum Operations Group Technology Roadmapping, Part 2. BioProcess Int. 15(2), 2017.
6 Scott C. Modern Laboratory Design. BioProcess Int. 12(11) 2014: 10–11.
7 Mirasol F. Automation Trend in Fill/Finish Reduces Contamination Risk. BioPharm Int. 31(6) 2018: 12–13.
8 Guide to Making Sense of Collaborative Robots. The Cobots Guide; https://cobotsguide.com.
9 Why Mobile Robots from Aethon; https://aethon.com/why-mobile-robots-from-aethon.
10 Owen-Hill A. Mobile Collaborative Robots: The Next Big Thing. The Robotiq Blog, 4 December 2018; https://blog.robotiq.com/mobile-collaborative-robots-the-next-big-thing.
11 Neo. Avidbots; www.avidbots.com.
12 Owen-Hill A. Ten Emerging Applications in Autonomous Logistics. The Robotiq Blog, 31 July 2018; https://blog.robotiq.com/10-emerging-applications-in-autonomous-logistics.
13 Dispensing Robot. Direct Industry; www.directindustry.com/industrial-manufacturer/dispensing-robot-75402.html.
14 Garfield L. MIT Students Invented a Robotic Kitchen That Could Revolutionize Fast Food. Business Insider, 18 April 2016; www.businessinsider.com/mit-students-invented-a-robotic-kitchen-2016-4.
15 Densford F. 11 Surgical Robotics Companies You Need to Know: Intuitive Surgical, Leading the Pack. MassDevice, 1 February 2017; www.massdevice.com/11-surgical-robotics-companies-you-need-to-know/2.
16 GE BioPark Biomanufacturing Campus: Virtual Tour; www.youtube.com/watch?v=cHHQaO49pfk.
17 Guillaume J-M. Developing Business Cases for New Products, New Technologies. Sanofi Pasteur: Lyon, France. 2011; https://biopharmservices.com/wp-content/uploads/2014/04/110607-SanofiPasteurBioSolve.pdf.
18 Decontamination Misting Tunnel. ViraTek: Sumerduck, VA; www.viratek.com/Misting%20Tunnel.html.
19 ViraTek Misting Tunnel in Operation. ViraTek: Sumerduck, VA; www.viratek.com/images/Images%202/August%201,%202005%20100.avi.
20 VivaCare Decontamination Misting Tunnels. Gelman Laminated Air Flow Systems: Singapore; https://gelmansingapore.com/product/vivacare-decontamination-misting-tunnels.
21 Sani Mister Misting Tunnels. Viking Medical: Medford Lakes, NJ; www.vikingmedical.com/sani-mister-misting-tunnel/.
22 Soft Robotic Grippers. Soft Robotics: Bedford, MA; https://www.softroboticsinc.com/flexible-packaging-solutions.
23 7000 RMS Microbial Detection Analyzer. Mettler Toledo: Billerica, MA; https://www.mt.com/us/en/home/products/Process-Analytics/Total-Organic-Carbon-TOC-analyzer/thornton-bioburden-analyzer.html.
24 Instantaneous Microbial Detection for Pharmaceutical Waters: IMD-W. BioVigilant: Tucson, NY; https://biovigilant.com/wp-content/uploads/2018/10/IMDW-Brochure02.20.17.pdf.
25 Instantaneous Microbial Detection Analyzer for Air: IMD-A. BioVigilant: Tucson, AZ; https://biovigilant.com/imd-a.
26 Denoya C. Implementation of Rapid Microbiological Methods: Some Technical Challenges Still Ahead. Amer. Pharm. Rev. 17 February 2014.
27 Tobe F. Why Co-Bots Will Be a Huge Innovation and Growth Driver for Robotics Industry. IEEE Spectrum 30 December 2015.
28 The Impact of Robots on Productivity, Employment, and Jobs: A Positioning Paper by the International Federation of Robotics. The International Federation of Robotics: Frankfurt, Germany, 2017; https://ifr.org/img/office/IFR_The_Impact_of_Robots_on_Employment.pdf.
29 Hagerty JR. Meet the New Generation of Robots for Manufacturing. The Wall Street Journal, 2 June 2015. https://www.wsj.com/articles/meet-the-new-generation-of-robots-for-manufacturing-1433300884.
30 Rozo L, et al. Special Issue on Learning for Human–Robot Collaboration. Autonomous Robots 42(5) 2018: 953–956; https://doi.org/10.1007/s10514-018-9756-z.
Corresponding author Rajesh G. Beri is vice president and head of CMC at a start-up biotech firm. Dave Wolton is biopharma technology consultant at PM Group. Carl-Helmut Coulon is head of future manufacturing concepts at Invite GmbH.