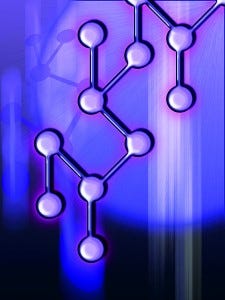
WWW.PHOTOS.COM
Many biotherapeutic proteins are naturally subject to aggregation. The clinical consequences of protein aggregation can be dramatic, not only affecting bioavailability and pharmacokinetics, but in extreme cases dramatically altering pharmacodynamics as well. Of equal or perhaps more importance is that aggregation is a principal source of unwanted immunogenicity in biotherapeutics. Aggregation-induced neutralizing antibodies and/or anaphylactic reactions are serious and growing US and European regulatory concerns. So they will have significant and growing influence on the future development and regulatory approval of both biosimilar and innovator biotherapeutics.
Surfactants are routinely used to prevent or limit protein aggregation. The most commonly used surfactants for this purpose are Tween 80 and Tween 20 polysorbates. They are both effective in preventing aggregation, but polysorbates are known to spontaneously oxidize to chemically reactive peroxides, epoxy acids, and aldehydes that can damage proteins and increase unwanted immunogenicity through creation of neoantigens (1).
Limitations of Polysorbates
Polysorbate surfactants are mixtures of fatty-acid esters of polyoxyethylene sorbitan with residual amounts of polyoxyethylene sorbitan, polyoxyethylene, and isosorbide. And as mentioned, they spontaneously form protein-damaging peroxides, epoxy acids, and reactive aldehydes (1). Polysorbates also hydrolyze in aqueous solution, releasing free fatty acids that can increase turbidity. Lot-to-lot variability in the concentration of chemically reactive species such as peroxides has been found to exceed an order of magnitude (2).
The US Food and Drug Administration (FDA) requires biosimilar developers to perform at least one clinical study that compares the immunogenicity of a proposed product with that of an approved reference product before submission for approval (3). The “Commercial Incentive” box describes additional concerns for such products.
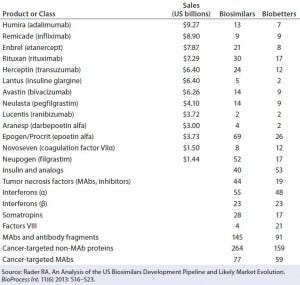
Table 1: Some major recombinant products and classes with biosimilars and biobetters currently in development; sales figures are given for original products where available.
Lot variability in polysorbate reactive contaminants can lead directly to lot variability in immunogenicity for innovator or biosimilar products. That can seriously complicate postmarket surveillance processes of the former as well as bioequivalence comparisons required for the latter’s regulatory approval. Although polysorbates are effective in reducing protein aggregation, the increased immunogenicity that can accompany that is a serious limitation. Equally effective alternatives that do not increase immunogenicity are clearly needed.

Table 2: Spontaneously formed chemically reactive oxidation products found in Polysorbate 80
Sources of Polysorbate-Induced Damage and Immunogenicity
Progressive oxidative damage to aminoacyl side chains on proteins can be caused by contaminating peroxides and other reactive molecular species from polysorbates. That can cause neoantigen formation over time, which increases or alters immunogenicity. Peroxides principally affect methionine and tryptophan moieties (4–9). Aldehydes react with primary amino groups on proteins and are known to induce immunogenicity in the absence of aggregation or adjuvants. Epoxy acids can react with all exposed nucleophiles (Table 2). Oxidative damage also leads to peptide-bond hydrolysis — and hence protein instability through alteration of a protein’s tertiary structure, which is yet another cause of aggregation (10).
As you might expect, manufacturers typically screen their sources of polysorbates to identify product lots with the lowest possible peroxide contamination. They reject lots that exceed established acceptable levels of contamination. The problem with this approach, however, is twofold: First, peroxide formation is spontaneous and progressive with any exposure to oxygen. Second, peroxides are not the only contaminants of concern. Polysorbates also contain epoxy acids and chemically reactive aldehydes, unreacted starting materials, and free fatty acids released through hydrolysis (causing increased turbidity). Therefore, product quality can be adversely affected as polysorbates undergo spontaneous changes in composition over time.
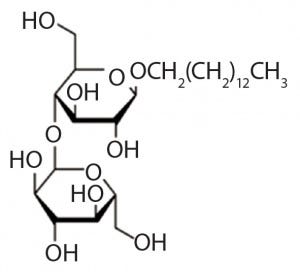
Figure 1: Structure of n-tetradecyl-beta-d- maltoside , the alkyl glucoside comprised of maltose and tetradecanol joined by a glycosidic bond
Alkylglucosides as Alternatives to Polysorbates
Alkylglucosides have been demonstrated to be effective alternatives to polysorbates in protecting proteins from aggregation. Certain alkylglucosides are highly effective in stabilizing peptide and protein biotherapeutics without causing the oxidative damage and unwanted immunogenicity associated with polysorbates (11–15). Alkylglucosides consist of a hydrophobic alkyl chain derived from a long-chain alcohol and a hydrophilic saccharide structure derived from glucose, linked together through a glycosidic bond (Figure 1). Unlike polysorbates, alkylglucosides do not oxidize to chemically reactive species such as peroxides.
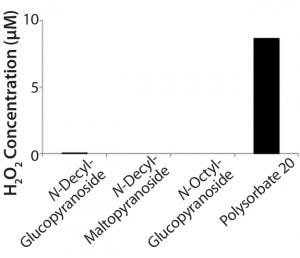
Figure 2: Hydrogen peroxide formation in solution from polysorbate 20 over a month at 40 °C compared with three alkyl glucosides; hydrogen peroxide was determined using the Amplex Red H2O2 assay (11).
Figure 2 compares formation of hydrogen peroxide in polysorbate 20 with the essentially complete lack of peroxide formation for three different alkyl glucosides. After storage of a 1% solution at 40 °C for one month, the hydrogen peroxide concentration approaches 10 mM in the polysorbate solution.
Alkylglucosides exhibiting surfactant properties typically have alkyl chains ranging from six to 18 carbon atoms, similar to most surfactants from other chemical categories. Alkyl monoglucosides contain a single glucose moiety; alkyl diglucosides contain a disaccharide comprising two glucose moieties linked through an interglycosidic bond (e.g., the disaccharide maltose). Some examples of potentially useful alkyl monoglucosides include hexyl-, octyl-, and decylglucoside. Examples of potentially useful alkyl diglucosides include octyl-, decyl-, dodecyl-, or tetradecyl maltoside.
Generally speaking, alkyl monoglucosides with chain lengths longer than 10 carbons have substantially reduced aqueous solubility. With a much larger hydrophilic structural component, alkyl diglucosides exhibit reasonable solubility for alkyl chain lengths up to about 14 carbon atoms. Beyond that, they have substantially reduced aqueous solubility. Nevertheless, even as aqueous solubility decreases with increasing alkyl chain length, solubility in more hydrophobic liquids increases. That makes larger alkyl diglucosides useful in pharmaceutically acceptable oil-based formulations such as those for oral softgels. Thus they have other drug applications apart from stabilization of biotherapeutics, most notably providing for greatly increased transmucosal absorption of drugs up to ~22 kDa in molecular weight (16–17).
Alkyl glucosides are designated “generally recognized as safe” (GRAS) substances for food purposes (18). Their simple structure allows for rapid metabolism into glucose and the corresponding long-chain alcohol (which is subsequently converted to the corresponding fatty acid) — both of which are normal components of food. As a result, the benign safety and toxicological properties of alkylsaccharides make them excellent potential candidates to consider for inclusion in biotherapeutic formulations.
Pharmaceutical-grade alkylglucosides are synthesized as single chemical species under good manufacturing practice (GMP) conditions. But to keep the manufacturing costs to a minimum, food-grade alkyl glucosides (used as emulsifiers and texturizers in many food products) are synthesized as mixtures of varying chain lengths from inexpensive sources of mixed alkyl chains such as coconut and palm oil. Tens of thousands of tons are manufactured annually to varying degrees of glucose moiety oligomerization. Coconut oil, for example, has alkyl-chain lengths ranging about 6–18 carbons, with the dominant species being carbon-12 and carbon-14 chain lengths. The average degree of glucose oligomerization in alkyl polyglucosides used for food purposes ranges ~1.2–1.4 glucose molecules. So they are mostly glucose with some di- and triglucose moieties such as maltose or maltotriose.
A Comparative Assessment
Aggregation of antibodies and other proteins is caused mainly by hydrophobic interactions that eventually lead to denaturation. When hydrophobic regions of a partially or fully unfolded protein are exposed to a hydrophilic aqueous environment, a decrease in entropy from structuring water molecules around the hydrophobic region forces the denatured protein to aggregate. That compromises its solubility, which can lead to precipitation and loss of activity.
Factors that contribute to aggregation in solution include protein concentration, temperature, pH, mechanical stress, freeze–thawing, oxidation, and light exposure. Forced degradation studies play a central role in therapeutic protein development. Protein concentration in a final drug product is dictated largely by its required therapeutic dose. Generally speaking, it is desirable to keep protein concentrations high and thus keep administration volumes as low as reasonably possible. That reduces infusion times and costs of administration while increasing patient convenience (19).
Proteins undergo varying degrees of degradation during purification and storage. Protein aggregation, physical stability, and immunogenicity are evaluated using a number of procedures (20). In the case of protein aggregation, typically a physical stress is introduced such as shaking or rotation at elevated temperatures. Damage from aggregation and unwanted immunogenicity can be assessed in different ways. One typical method is to measure turbidity under both static and continuous-agitation conditions. As proteins undergo substantial aggregation, their solutions become more turbid. That can be quantitated with absorbance spectroscopy or light-scattering measurements. Lower levels of aggregation (e.g., dimers and trimers) often go undetected by simple turbidimetric methods. However, they may be easily detected using analytical size-exclusion chromatography (SEC). Samples are typically withdrawn at regular intervals and SEC analyzed for monomer percentage.
Immunogenicity assessment for biotherapeutics is considerably more complicated and multidimensional. In recent years, a number of articles have been published about the underlying philosophical approach to making meaningful determinations (21–26). Wadhwa and Thorpe provide an excellent summary, detailed description, and analysis of the pros and cons of well-accepted screening assays for antibody detection (26). Useful methods include direct enzyme-linked immunosorbent assays (ELISAs), bridging ELISAs, electrochemiluminescence assays, radioimmunoprecipitation assays, and surface-plasmon resonance analysis. A growing number of reports concern additional methods of detecting and assessing antibody response based on gene expression (27–31). At present, no single methodology stands out as a “gold standard” for immunogenicity assessment, so a combination of techniques should be incorporated in a multitiered detection approach.
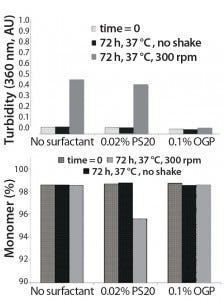
Figure 3: Comparative study regarding stabilization of an anti-CD11α monoclonal antibody by polysorbate 20 (PS 20) and octyl glucoside (OGP)
It is useful to examine some examples off the effects of polysorbates and alkyl glucosides. Figure 3 illustrates the results of a comparative study on stabilization of an anti-CD11α monoclonal antibody (MAb) using 0.02% polysorbate 20 (PS20) and 0.1% octyl glucoside (OGP) compared with a no-surfactant control in both static and constant-agitation conditions (shaking at 300 rpm for 72 hours) at 37 °C. Turbidimetric results (top) and assessment of the degree of remaining monomer (bottom) indicate that octylglucoside effectively maintains the monomer percentage of the antibody with shaking compared with an unshaken sample stored under similar conditions. PS20 appears to be significantly less effective (11).
Oxidative Damage: Oxidation (including that induced by light) is a major degradation pathway affecting protein stability and potency through modification of aminoacyl residues, hydrolysis of peptide bonds, and alteration of a protein’s tertiary structure (10, 32–35). Numerous protein oxidation reactions have been reported. In alkaline or neutral media, the aminoacyl residues of cysteine, histidine, methionine, tryptophan, and tyrosine are especially prone to oxidation. Methionine is sensitive to oxidation in acidic conditions, and some aminoacyl residues (e.g., tryptophanyl residues) undergo oxidation as a result of exposure to light. Table 2 summarizes the specific aminoacyl residues affected by oxidation. Determining the extent of chemical modification from oxidative processes is somewhat complicated, requiring measurement of final oxidation products formed at individual aminoacyl residues within a protein sequence.
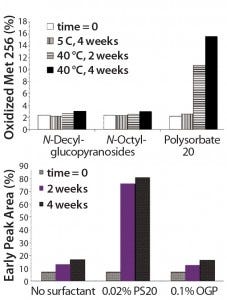
Figure 4: (top) Methionine oxidation in an anti-CD11 monoclonal antibody arising from intrinsic polysorbate contaminants; (bottom) effect of different alkylglycosides or polysorbate 20 in preventing oxidation of an anti-CD22 monoclonal antibody in aqueous solution (11)
Figure 4 shows (top) the effects of different alkylglycosides and polysorbate 20 in preventing methionine oxidation in an antiCD11α MAb in aqueous solution and (bottom) the effect of different alkylglycosides or polysorbate 20 on oxidation prevention for an anti-CD22 MAb in aqueous solution (11). No significant increase in the percentage of oxidized Met256 (Figure 4, top) was observed in solutions containing decylglucoside or octylglucoside following storage at 40 °C over four weeks compared with a baseline level of ~2% oxidation of the same amino acid residue at time zero. By contrast, ~16% of the Met256 residues were oxidized in the PS20-containing anti-CDllα solutions. Thus, alkyl glycosides exhibit a significant advantage over polysorbates as proteinstabilizing agents in aqueous formulations.
The bottom panel of Figure 4 shows the effect of various alkylglycosides or PS20 on preventing oxidation of an anti-CD22 antibody in aqueous solution. Oxidation of methionine and tryptophan in an anti-CD22 MAb was studied using iron-catalyzed hydrogen peroxide (Fenton reagent). Solutions containing 0.1 ppm Fe and 1 ppm hydrogen peroxide were stored for two or four weeks at 40 °C, either in the absence of surfactant or in the presence of PS20 or octyl glucoside. The percent oxidation of methionine and tryptophan residues was determined from the early peak area percentage of the antibody eluting through a phenyl reverse-phase high-performance liquid chromatography (RP-HPLC) column. Note that Fenton-reagent–induced oxidation of both residues in the anti-CD22 MAb is inhibited in solutions containing octyl glucoside but not in the no-surfactant control solution or in those containing PS20.
Prevention of Light-Induced Oxidation: Alkylglucosides prevent light-induced oxidation of tryptophan residues in biotherapeutics in aqueous solution. Esue and Sharma analyzed the effect of different surfactants on light-induced oxidation of tryptophan residues in a humanized anti-oxidized low-density lipoprotein (LDL) MAb after 24-hour exposure in a light box (11). The surfactants included four alkylglucosides (hexyl glucoside, hexyl maltoside, dodecyl glucoside, and dodecyl maltoside) and polysorbate 80. The extent of oxidation was measured using HPLC with mass spectrometry following reduction, alkylation, and trypsin digestion of the MAb samples. In general, the alkylglycosides appeared to convey a significant degree of protection against light-induced oxidation. Similar results for hexyl glucoside and hexyl maltoside also have been reported by other authors (36). Dodecyl maltoside appears to be the most effective nonionic surfactant in preventing light-induced oxidation of tryptophan, whereas polysorbate 80 was least effective at similar concentrations (0.19 mg/mL and 0.2 mg/mL).
A Promising Alternative
Many biotherapeutic proteins are naturally subject to aggregation, resulting in reduced efficacy and development of unwanted immunogenicity. Aggregation-induced neutralizing antibodies and anaphylactic reactions are serious and growing concerns of both the FDA and the European Medicines Agency (EMA). This will have significant and growing impact on development and regulatory approval of both biosimilar and innovator biotherapeutics. Surfactants are used routinely to prevent or limit aggregation and make higher-concentration biotherapeutics feasible. The most commonly used surfactants for that purpose are Tween 80 and Tween 20 polysorbates.
Although they are highly effective in preventing protein aggregation, polysorbates do spontaneously oxidize to chemically reactive peroxides, epoxy acids, and aldehydes — all of which can damage proteins and increase unwanted immunogenicity through creation of neoantigens. Alkylglucosides are in development at both major pharmaceutical and small biotech companies as potential replacements. Alkylglucosides are as effective as (and in some cases more effective than) polysorbates in preventing protein aggregation without their oxidative damage problems. Polysorbates are mixtures of several chemical species with different molecular weights and some contaminating unreacted chemical reactants and damaging oxidative species. All of those vary among lots. By contrast, alkylglucosides are single molecular chemical species with no lot variability in composition that are easy to characterize for quality control (QC), quality assurance (QA), and overall manufacturing. Thus far, alkylglucosides appear to address the shortcomings of polysorbates without exception. Although biotherapeutics incorporating alkylglucosides are in development, none have yet been approved by the FDA or EMA.
Biotherapeutics incorporating alkylglucosides rather than polysorbates are likely to achieve substantial commercial benefits. Because immunogenicity can reduce or eliminate a protein drug’s efficacy upon subsequent administration — and may even neutralize a patient’s corresponding residual biological activity — it would be difficult if not unethical to justify using a product demonstrated to be immunogenic over one with less or no immunogenicity. Incorporating alkylglucosides in place of polysorbates for innovator or biosimilar biotherapeutics currently in development could provide clinically superior products, resulting in a strong and differentiable market franchise.
References
1 Maggio ET. Biosimilars, Oxidative Damage, and Unwanted Immunogenicity. BioProcess Int. 11(6) 2013: 28–34.
2 Wasylaschuk WR, et al. Evaluation of Hydroperoxides in Common Pharmaceutical Excipients. J. Pharm. Sci. 96(1) 2007: 106–116.
3 CBER/CDER. Scientific Considerations in Demonstrating Biosimilarity to a Reference Product: Guidance for Industry. US Food and Drug Administration: Rockville, MD, April 2015; www.fda.gov/downloads/Drugs/GuidanceComplianceRegulatoryInformation/ Guidances/UCM291128.pdf.
4 Ji JA, et al. Methionine, Tryptophan, and Histidine Oxidation in a Model Protein, PTH: Mechanisms and Stabilization. J. Pharm. Sci. 98, 2009: 4485–4500.
5 Simat TJ, Steinhart H. Oxidation of Free Tryptophan and Tryptophan Residues in Peptides and Proteins. J. Agric. Food. Chem. 46, 1998: 490–498.
6 Thliele GM, et al. Soluble Proteins Modified with Acetaldehyde and Malondialdehyde Are Immunogenic in the Absence of Adjuvant. Alcohol Clin. Exp. Res. 22, 1998: 1731–1739.
7 Moghaddam AE, et al. Reactive Carbonyls Are a Major Th2-Inducing Damage-Associated Molecular Pattern Generated By Oxidative Stress. J. Immunol. 187, 2011: 1626–1633.
8 Allison ME, Fearon DT. Enhanced Immunogenicity of Aldehyde-Bearing Antigens: A Possible Link Between Innate and Adaptive Immunity. Eur. J. Immunol. 30, 2000: 2881–2887.
9 Kiyoharaa S, et al. Amino Acid Addition to Epoxy-Group-Containing Polymer Chain Grafted onto a Porous Membrane. J. Membrane Sci. 109(1) 1996: 87–92.
10 Davies KJ. Protein Damage and Degradation By Oxygen Radicals: I, General Aspects. J. Biol. Chem. 262, 1987: 9895–9901.
11 Esue O, et al. Compositions and Methods for Stabilizing Protein-Containing Formulations. US Pat. Appl. 20130224213, 29 August 2013.
12 Mohadeseh T, et al. The Effects of Dodecyl Maltoside and Sodium Dodecyl Sulfate Surfactants on the Stability and Aggregation of Recombinant Interferon Beta1b. J. Interfer. Cytokine Res. 34 (11) 2014: 894–901.
13 Maggio ET. Stabilizing Alkylglycoside Compositions and Methods Thereof. US Pat. No. 8,226,949, 24 July 2012.
14 Maggio ET. Stabilizing Alkylglycoside Compositions and Methods Thereof. US Pat. No. 8,846,044, 30 September 2014.
15 Rifkin RA, et al. N-Dodecyl-β-dMaltoside Inhibits Aggregation of Human Interferon-β-1b and Reduces Its Immunogenicity. J. Neuroimmune Pharmacol. 6(1) 2011: 158–162.
16 Maggio ET, et al. High Efficiency Intranasal Drug Delivery Using Intravail® Alkylsaccharide Absorption Enhancers. Drug Deliv. Transl. Res. 3(1) 2013: 16–25.
17 Illum L. Nasal Drug Delivery: Recent Developments and Future Prospects. J. Contr. Rel. 161(2) 2012: 254–263.
18 EPA. Alkyl (C10-C16) Polyglycosides: Exemptions from the Requirement of a Tolerance. Fed. Regist. 70(177) 2005: 54281– 54286.
19 Philo JS, et al. Mechanisms of Protein Aggregation. Curr. Pharmaceut. Biotechnol. 10, 2009: 348–351.
20 Maggio ET. Use of Excipients to Control Aggregation in Peptide and Protein Formulations. J. Excip. Food Chem. 1(2) 2010: 40–49.
21 Wadhwa M, et al. Strategies for Detection, Measurement, and Characterization of Unwanted Antibodies Induced By Therapeutic Biologicals. J. Immunol. Meth. 278, 2003: 1–17.
22 Wadhwa M, et al. Strategies and Assays for the Assessment of Unwanted Immunogenicity. J. Immunotoxicol. 3, 2006: 115–121.
23 Rosenberg AS, et al. A Risk-Based Approach to Immunogenicity Concerns of Therapeutic Protein Products: Part 1 — Considering Consequences of the Immune Response to a Protein. BioPharm Int. 17, 2004: 22–26.
24 Wadhwa M, Thorpe R. The Challenges of Immunogenicity in Developing Biosimilar Products. IDrugs 12(7) 2009: 440– 444.
25 Stas P, et al. Strategies for Preclinical Immunogenicity Assessment of Protein Therapeutics. IDrugs 12(3) 2009: 169–173.
26 Wadhwa M, et al. Unwanted Immunogenicity: Lessons Learned and Future Challenges. Bioanalysis 2(6) 2012: 1073–1084.
27 Wei X, et al. Development and Validation of a Cell-Based Bioassay for the Detection of Neutralizing Antibodies Against Recombinant Human Erythropoietin in Clinical Studies. J. Immunol. Meth. 293, 2004: 115–126.
28 Bertolotto A, et al. Development and Validation of a Real Time PCR–Based Bioassay for Quantification of Neutralizing Antibodies Against Human Interferon-B. J. Immunol. Meth. 321(1–2) 2007: 19–31.
29 Moore M, et al. Measurement of Neutralising Antibodies to Type I Interferons By Gene Expression Assays Specific for Type 1 Interferon-Inducible 6–16 mRNA. J. Pharm. Biomed. Anal. 49, 2009: 534–539.
30 Lallemand CJ, et al. Quantification of Neutralizing Antibodies to Human Type I Interferons Using Division-Arrested Frozen Cells Carrying an Interferon-Regulated Reporter-Gene. J. Interferon Cytokine Res. 28, 2008: 393–404.
31 Kropshofer H, Singer T. Overview of CellBased Tools for Pre-Clinical Assessment of Immunogenicity of Biotherapeutics. J. Immunotoxicol. 3(3) 2006: 131–136.
32 Davies KJ, et al. Protein Damage and Degradation By Oxygen Radicals: II, Modification of Amino Acids. J. Biol. Chem. 262(20) 1987: 9902–9907.
33 Nguyen TH. Chapter 4: Oxygen Degradation of Protein Pharmaceuticals. Formulation and Delivery of Protein and Peptides (ACS Symposium Series), 1st Edition. Cleland JL, Langer R, Eds. American Chemical Society: Washington, DC, 23 July 2009; 59–91.
34 Hovorka S, et al. Oxidative Degradation of Pharmaceuticals: Theory, Mechanisms and Inhibition. J. Pharmaceut. Sci. 90(3) 2001: 253–269.
35 Li S, et al. Chemical Instability of Protein Pharmaceuticals: Mechanisms of Oxidation and Strategies for Stabilization. Biotechnol. Bioeng. 48, 1995: 490–500.
36 Adem YT, et al. Hexyl Glucoside and Hexyl Maltoside Inhibit Light-Induced Oxidation of Tryptophan. J. Pharmaceut. Sci. 103(2) 2014: 409–416.
Edward T. Maggio, PhD, is president and chief executive officer at Aegis Therapeutics LLC, 11770 Bernardo Plaza Court, Suite 353, San Diego, CA 92128; 1-858-618-1400, fax 1-858618-1441, mobile 1-858-967-6840; [email protected].