Implementing a Fully Disposable MAb Manufacturing Facility
Biopharmaceutical contract services have seen some very important changes and growth in recent years, part of an increasing trend toward outsourcing by pharmaceutical companies both large and small. And we have seen a significant change in the types of services demanded by clients. In addition to typical services such as validation, analytical services, and training, for example, demand has increased for outsourcing of process development, mammalian cell culture, good manufacturing practice (GMP) production, and final formulation and filling. Those demands have presented a substantial challenge for contract service companies to provide integrated services together with flexible and adaptable facilities for multiproduct capability in both upstream and downstream processing. As always, timelines are expected to be as short as possible, with rapid product changeover, effective cleaning procedures, and lowest possible costs.
PRODUCT FOCUS: ALL BIOLOGICS
PROCESS FOCUS: MANUFACTURING
WHO SHOULD READ: PROCESS DEVELOPMENT AND FACILITIES/OPERATIONS
KEYWORDS: MONOCLONAL ANTIBODIES, CELL CULTURE, HARVEST, PURIFICATION, DISPOSABLES, ENGINEERING
LEVEL: INTERMEDIATE
Photo 1:
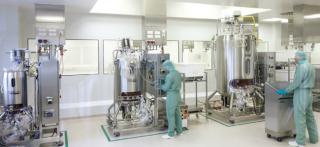
Photo 1: ()
For those reasons, contract service organizations have to continually review their operations to enhance their capabilities and remain competitive. For example, any advances in process equipment could significantly affect changeover times, flexibility, manufacturing capacity, sterility assurance, costs, and so on. Here, we report on a project that resulted in successful development and implementation of a fully disposable, single-use (SU) process for generic antibody manufacturing, incorporating both upstream and downstream processing. We describe the specific benefits over our traditional stainless-steel systems and remaining challenges to be addressed for wider implementation in our facility.
Background
Rentschler is a contract services company that provides flexible, integrated services from one source. Although its history in pharmaceutical manufacturing goes back as far as 1927, a focus on biotechnology took place 20 years later with the development of veterinary vaccines. In 1983, Rentschler was the first company to obtain market approval for a natural b-interferon compound (Fiblaferon) followed in 1989 by a recombinant IFN-γ interferon and topical IFN-b gel.
In 1993, all biotech activities were consolidated into Rentschler Biotechnologie GmbH, a contract development and manufacturing organization offering full services in development and production of biopharmaceuticals. Subsequent investments have led to a facility with bioreactor capacities up to 2,500 L and nine state-of-the-art suites for GMP production as well as three GMP filling lines and more than 600 fully trained employees.
Those facilities have been successfully used for manufacture of various recombinant biotechnology proteins as well as antibody products for many clients. But Rentschler recognized that introducing SU systems could potentially offer significant benefits over our stainless steel upstream and hybrid systems downstream. Rentschler therefore embarked on a concept study to assess the suitability of its processes for conversion to a complete SU system. Afterward, the company prepared and proceeded with a project plan for implementing a single-use upstream and downstream process for generic antibody manufacture.
SU Manufacturing Concept Study
Rentschler’s concept study focused on development of two 1,000-L manufacturing lines in an existing building with fully disposable upstream and downstream unit operations. In 2010, the company successfully implemented a single-use bioprocessing line as part of its development plan. The concept study identified a number of beneficial features, as listed in the “Single-Use Benefits” box.
The concept study showed that many restrictions inherent to stainless steel systems could be removed by replacing them with SU technology. Results also suggested that successful implementation would require reconstructing part of an existing building. Also, many of Rentschler’s clients were specifying disposable technologies. So it was clear that SU manufacturing systems should be a fundamental part of Rentschler’s business strategy for the future.
Existing Process for MAb Manufacture
Rentschler’s SU development program was based on the process diagrammed in Figure 1. With a working capacity of ≤3,000 L, the process was based on a hybrid system using mainly stainless steel, cleanable equipment typically associated with MAb production but some SU components in downstream processing. As a contract manufacturer, Rentschler’s requirement for multiproduct capabilities for each line demand thorough and fully validated CIP/SIP procedures. Those were especially important to eliminate cross-contamination and the need for dedicated lines when processes use media containing animal components in their seed trains.
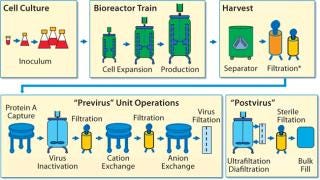
Figure 1: ()
Additional checks were needed for performance and integrity after repeated cleaning and regeneration of reusable components such as ultrafiltration (UF) cassettes, ion-exchange chromatography resins, and virus filters. All these and other factors (described below) were important drivers in evaluating SU technology.
System for Comparative Studies and PQ
A single-use option was considered for all the unit operations shown in Figure 1 except the centrifugal separator, which Rentschler could eliminate by developing an enhanced, SU depth filtration system. Figure 2 shows the sequence of SU unit operations for performance qualification (PQ), which was a part of the platform process for the total system. For initial comparative and PQ studies, we did not include the protein A step.
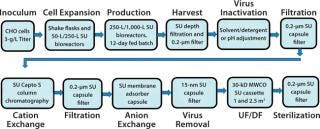
F
igure 2: ()
We chose to study 200-L and 1,000-L bioreactors to complement our portfolio range, from 30 L through 250 L and 500 L up to 2,500 L. The new facility also can operate 2,000-L SU bioreactors. It is not possible to give a detailed account of all studies on all unit operations here. Instead, we offer a brief summary for some of them and a detailed description of others to show how SU systems performed in a given process.
Single-Use Benefits
Fast Implementation of Processes: Lead times for process equipment could be substantially shortened with single-use components, typically four to six months for skids.
Reduced Capital Expenditure: Eliminating stainless-steel processing equipment and storage tanks could substantially lower capital expenditures.
Reduction of Project Cycle Times: Product changeover times could be reduced (e.g., short set-up time with prefabricated single-use systems), giving increased production capacity and shorter lead times for clients. Clean-and steam-in-place (CIP/SIP) are reduced when using presterilized systems, as is maintenance of upstream and downstream equipment.
Increased Flexibility: Maximum flexibility comes with use of suites and equipment such as plug-and-play skids, cell lines (e.g., CHO, hybridomas), and animal components (e.g., bovine serum) if necessary. This eliminates need for dedicated product-specific lines to prevent cross-contamination (e.g., media containing animal-derived components and cells). And it allows for efficient scale-up from a client’s own single-use development systems.
Cell Culture: After initial comparative studies of bioreactors from three different manufacturers, we selected the Xcellerex XDR bioreactor with bottom-mounted, magnetically driven three-pitched blades and sintered disks or drilled-hole spargers. Using Chinese hamster ovary (CHO) cells expressing a monoclonal antibody (MAb) in a high-density fed-batch process (inoculum cell concentration >20 × 106/mL) with chemically defined media, we saw excellent performance in cell viability and concentration, product formation, and quality.
Within Rentschler’s production capabilities, the new systems were integrated by measuring relevant parameters of bioprocess technology such as mixing times, oxygen mass-transfer coefficients, volumetric power input, and heat-transfer rates. Overall, the new equipment gave comparable results for cell viability and concentration, product formation, and other relevant metabolite parameters from bench-top to subsequent 200-L and 1,000-L bioreactors.
Harvest and Fluid Clarification: Rentschler’s studies on SU depth filtration as an alternative to a disposable centrifugal separator are published in detail elsewhere (1). Results showed that low turbidity, high product recovery, and good throughput could be achieved by feeding biomass directly from bioreactors into depth filters. The highest throughput and lowest costs came with a single-stage disposable Pall Stax PDK6 capsule filter followed by a 0.2-µm Pall Supor EKV membrane capsule filter. Other benefits included robust performance (no breakthrough) and good scalability.
Rentschler has completed further studies of SU depth filters to evaluate the effects of biomass sedimentation before filtration. Figure 3 is a diagrammatic representation of the results. In this study, a two-stage depth filtration system was used (Pall Stax PDK and PDD capsules) followed by a 0.2-µm Pall Supor EKV pleated membrane filter. The CHO cell biomass from a 1,000-L bioreactor was divided equally into two containers. One 500-L batch then passed directly into the filtration system, and the second batch was allowed to sediment for five hours at 10 °C and then filtered.
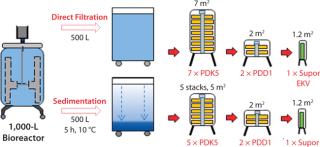
Figure 3: ()
We monitored pressure upstream of the filters as well as flow rate (flux) and throughput (harvest weight). We also recorded viable cell count, initial biomass content, turbidity at the start and end of filtration, and product titer. When pressure upstream of the first depth filter (PDK5) approached its maximum limit of 1.0 bar, the filter’s capacity was reached. To maximize product recovery, we then passed 50 L of phosphate buffered saline (PBS) through the filter system, followed by an air blow-out to recover residual liquid.
The total viable cell count for each filtration run was ~20 × 106 with a wet biomass concentration of 8% and a product titer of 4 g/L. Results of direct biomass filtration (Figure 4) are the following:
Pressure upstream of the first depth filter (PDK5) rose steadily to reach the 1-bar maximum when 350 kg of biomass had been filtered.
Pressures for depth and membrane filters downstream showed much smaller increases, indicating good protection by the PDK5 filter.
Biomass turbidity of ~1,200 NTU before harvest was reduced to an acceptable level of 11 NTU after harvest.
Product recovery was good at 92% of the preharvest loading (1,625 g).
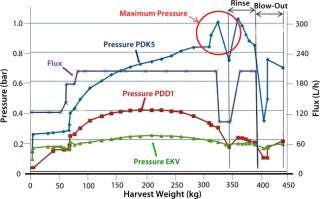
Figure 4: ()
Figure 5 charts the results of biomass filtration after sedimentation. These showed some significant differences from the direct-filtration data:
The first depth filter (PDK5) showed a much more gradual increase in pressure, indicating improved filterability of the biomass.
Throughput significantly increased from 350 kg to 450 kg despite a decrease in the PDK5 filter size from seven (7 m2) to five (5 m2) lenticular discs. This represented a 50% increase in filter capacity by unit area from 44 L/m to >66 L/m.
When 450 kg of biomass was harvested, the residual high-solids sediment was then filtered. Pressure increased as expected to the 1-bar limit. We then applied PBS rinsing and filter blow-out with air to ensure good product recovery.
Pressure profiles of the depth and membrane filters downstream were unchanged, again confirming appropriate protection by the PDK5 filter. The postharvest turbidity value was acceptable at 9 NTU, and product recovery was high at 91% of the preharvest loading (1,915 g).
x;margin-top:5px;cursor:pointer;” >
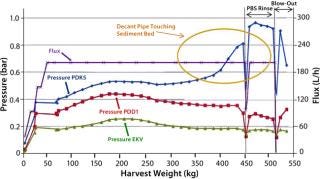
Figure 5: ()
Those results showed that throughputs achieved in our previous studies (1) could be significantly increased by allowing suspended solids to settle for five hours before filtration. The benefits of this additional sedimentation step come in two ways: filter-size reduction to lower disposables costs, improve system handling, and minimize product loss in filter media; and an increase in biomass batch size for the same-size filter.
That study also confirmed our previous conclusions that SU depth filtration can provide a practical and robust alternative to mechanical separators for cell harvest in MAb manufacturing. The main factors influencing throughput and economics are the quantity and properties of the biomass. Optimization of the filter train with further advances in filter media could provide additional benefits in throughput, filter size, and scale-up.
Ion-Exchange (IEX) Chromatography: Only a brief summary of the IEX stages is presented here.
Cation Exchange: For this unit operation, a GE Healthcare Capto-S resin IEX column was retained as a single-use application with a manually packed column. Bed volume was 25.5 L, with a bed height and diameter of 26.5 and 35 cm. Maximum allowed before-and after-column pressures were 5 bar and 3 bar, respectively. We used a typical capture and pH elution protocol with flow rates up to 510 L/h and linear flow rates of 300 cm/h reduced to 100 cm/h during elution. And we continually monitored ultraviolet (UV) absorbance, pH, and conductivity.
Photo 2:
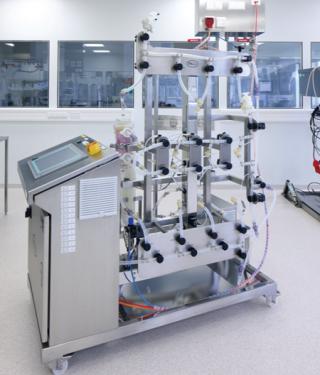
Photo 2: ()
Anion Exchange: For this unit operation, we replaced column chromatography with a Sartobind Q single-use membrane adsorber capsule from Sartorius Stedim Biotech (5.94-m2 membrane area, 500-L/h flow rate). We used the same controller as for the cation-exchange process.
Virus Removal: Membrane filtration for viral clearance has been an integral part of MAb processes for many years. Hollow-fiber membrane cartridges are used in single-pass, direct-filtration mode, sometimes called dead-end filtration. Advanced surface-modified, hydrophilic membranes with high void volume and precise fine-pore structure ensure minimum fouling and high viral titer reduction.
Photo 4:
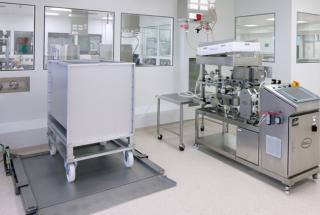
Photo 4: ()
For this stage, we had Pall Life Sciences develop a purpose-designed automated skid for viral filtration (Photo 2) with the following features: robust operation with minimal risk of operator error, precise control over parameters such as pressure and flow rate, built-in integrity testing, and flexibility for different processes and filtration modules. All types of virus filters can be mounted (e.g., 1–16 m2 of membrane area with Planova 20N/15N modules from Asahi Kasei Ltd.). Manifold and filter assembly is easy, fast, and robust using sterile connectors. With a Siemens S7 control system (Win CC visualization), the system is applicable to depth filtration and 0.2-µm filtration as well.
Ultrafiltration (UF) and Diafiltration (DF): The post-virus stage of our process requires antibody purification through ultrafiltration (UF) and diafiltration (DF). This is followed by final retentate concentration before sterile filtration and bulk storage.
We evaluated UF cassettes from three suppliers using an automated Pall UF skid coupled with single-use manifolds (Photos 3 and 4, Figure 6). As with viral filtration, introduction of automation into this unit operation provided an innovative approach to guarantee robustness, precise process control, and flexibility for multiproduct manufacturing. Table 1 lists further details and test parameters. Based on our comparative results (see above), we selected the Pall membrane system for more detailed studies. Other published studies on this type of UF system also show its performance to be suitable for SU applications in biomanufacturing (2).
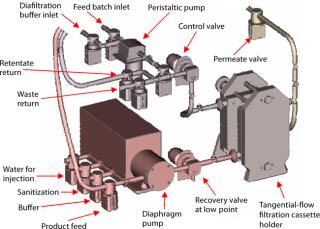
Figure 6: ()
Table 1: Single-use ultrafiltration test parameters (test membranes supplied by Pall, Novasep, and Sartorius Stedim Biotech)
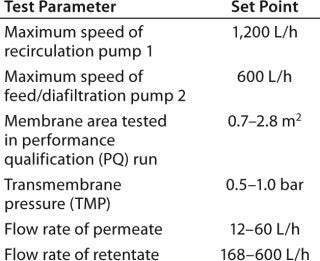
Table 1: Single-use ultrafiltration test parameters (test membranes supplied by Pall, Novasep, and Sartorius Stedim Biotech) ()
We evaluated two filter areas (0.7 m2 per 1 m2 and 2.5 m2 per 2.8 m2) in our final qualification studies to assess both performance and scalability. To calculate the diafiltration and concentration factors, we recorded volumes of feed, retentate, and permeate.
In qualification studies, permeate flow rates showed no major reduction at constant transmembrane pressure (TMP) and retentate flow rates, indicating an absence of significant membrane fouling. Total processing time for this stage was just over three hours. Results presente
d in Table 2 can be summarized as follows: More than five diafiltration volumes could be passed through the system. Initial and final volume-concentration factors of 11.5 and 1.7 were achieved. and results for the two membrane areas showed appropriate scalability.
Table 2: Ultrafiltration and diafiltration performance qualification
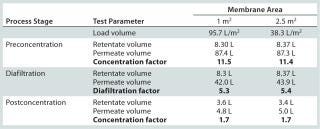
Table 2: Ultrafiltration and diafiltration performance qualification ()
Sterile Filtration and Bulk Storage: Our final process stage incorporates a 0.2-µm sterilizing-grade Pall Supor EKV SU capsule filter, then sterile transfer into a flexible biocontainer.
Reconstruction of Existing Building: Rentschler’s plan for a fully disposable upstream and downstream process at 1,000-L scale required a customized manufacturing area. The company reconstructed an existing building as shown in Figure 7. The main areas are two upstream processing (USP) zones for 1,000-L SU bioreactors, previrus and postvirus downstream processing (DSP) zones, a separate inoculum zone, storage and preparation areas, and in-process control (IPC) laboratory (e.g., pO2, pCO2, pH, cell count, viability, sodium, potassium, glucose, and so on).
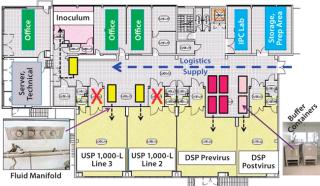
Figure 7: ()
Important considerations included logistics and material flow and flexibility in use of manufacturing lines. SU components allowed us to restrict movement of materials and people into our cleanroom suites for inoculum, USP, and all DSP processes. To do so, we located all bulk buffer and media in biocontainers (colored rectangles) in the central supply corridor. Fluid was transferred (dotted lines) through piped manifolds sealed into the partition walls instead of by moving bulk containers into pressure-controlled suites through air-locked doorways (as shown by a red cross). That simplified fluid handling, reduced operator entry into cleanroom areas (and concomitant risk of cross-contamination), and lessened demand on our heating, ventilation, and air-conditioning (HVAC) systems.
Timelines and Capital Expenditure: The need to reconstruct an existing building imposed challenges to our project timeline. But manufacturing schedules and capacity were not severely impaired. Strict capital expenditure targets were set. Figure 8 shows the project stages and timings achieved. The total project duration from start to completion (ready for the first customer project) was only 18 months. Capital expenditure was also within budget and much lower than investment costs for an equivalent reusable system based on stainless steel.
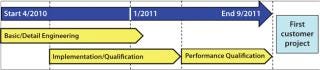
Figure 8: ()
Involvement of Suppliers: Another key element in the success of this project was establishing a close working relationship with suppliers and manufacturers of disposable equipment. For example, Rentschler involved specialists from Pall Life Sciences to assist in design, development, and qualification of the process and to ensure that the system was engineered in accordance with good engineering practice (GEP) (3). This collaborative approach ensured that a system best suited to our process was developed and that timelines were met.
Process Benefits and Remaining Challenges
The potential benefits identified in our concept study were realized in practice. In quantitative terms, manufacturing capacity increased by 20%, and fixed costs were reduced by 40% due to lower depreciation, less maintenance, and dilution of fixed costs. Energy costs were also significantly lower. Raw-material costs increased as expected, the major contributor being chromatography media (~60%).
Having achieved its initial objective of developing and operating two 1,000-L USP and DSP single-use processes, Rentschler is now addressing other challenges for wider implementation, especially at production scales of 2,500 L and greater. This next phase will involve several challenges and restrictions:
Cell harvest (disposable centrifuge for production scale, depth filter capsules for large amounts of biomass)
Flexible biocontainers (a practical preuse leak test performed on site)
Chromatography and tangential-flow filtration (TFF) systems (limited in size and scale-up)
Handling of disposable materials (manual operations require rigorous standard operating procedures, SOPs, and continuous operator training; further availability of automated skids could be beneficial, as could more robust materials to minimize the risk of damage)
Leachables and extractables (additional process-specific data)
Sensors and optical probes (a wider range of robust disposable probes).
Proof of Concept
This project progressed from an initial concept study through a design and development phase to the final implementation of a single-use USP and DSP system. It was the first SU process for antibody production to be operational on a 1,000-L production scale in the biomanufacturing industry. Close cooperation with SU system manufacturers facilitated design, development, and qualification of the system. A performance qualification run was successfully completed in a customized manufacturing suite for both USP and DSP operations. And scheduled targets for timeline and capital investment were achieved.
An innovative approach using automated skids for virus filtration and TFF enabled us to introduce SU technology into both critical and complex unit operations while retaining strict process control. With this innovative approach, our Rentschler Biotechnologie site in Laupheim, Germany, received the ISPE’s 2012 Facility of the Year Award in the equipment innovation category. Rentschler is very proud to be a category winner of this distinguished award.
Special attention was focused on operator training and SOPs to achieve a successful implementation. The single-use system enabled Rentschler to introduce a flexib
le approach in the use of cleanrooms and equipment, increasing manufacturing capacity and eliminating the need for dedicated product-specific lines. Significant cost savings and other benefits have also been achieved. The project has confirmed that SU manufacturing is feasible for MAb processes at a 1,000-L scale.
About the Author
Author Details
Benjamin Minow ([email protected]) is director of cell culture disposable manufacturing, and corresponding author Peter Rogge ([email protected]) is director of protein chemistry, Rentschler Biotechnologie GmbH, Erwin-Rentschler-Straße 21, 88471 Laupheim, Germany; 49-7392-701-0, fax 49-7392-701-300. Kevin Thompson is European group manager for PASS at Pall Life Sciences, Europa House, Havant Street, Portsmouth, Hampshire PO1 3PD, UK; 44-23-9230-3303, fax 44-23-9230-2506.
REFERENCES
1.) Pegel, A. 2011. Evaluating Disposable Depth Filtration Platforms for MAb Harvest Clarification. BioProcess Int. 9:52-56.
2.) Nicholson, P, and E. Storm. 2011. Single-Use Tangential-Flow Filtration in Bioprocessing. BioProcess Int. 9:38-47.
3.) Botterill, M, and B. Rawlings. 2008. Applying Good Engineering Practices to the Design of Single-Use Systems. BioProcess Int. 6:18-25.
You May Also Like