May 1, 2009
Vaccines have been around a long time — longer than any other biologic medical products. Since the 1700s, when a British doctor inoculated people against smallpox using Variolae vaccinae (cowpox virus), we’ve referred to such immunizing treatments as “vaccines.” Most children in developed countries grow up knowing there will be occasional “vaccinations,” usually injections, required to get into school and stay there (which may or may not seem like a great thing, depending on who you talk to). Similarly, people from developed nations traveling to underdeveloped ones expect to undergo certain vaccinations to prevent diseases that they would most likely not be exposed to at home. In the 21st century, vaccines are a routine part of our lives.
All the earliest vaccines were made using animal cell culture — some in petri dishes, some in diseased animals or humans — which produced large numbers of wild-type viruses or bacteria that could be weakened, killed, or sometimes used live as vaccines. Bacterial or toxoid vaccines were developed during the 20th century, and these were mostly created from weakened toxins. Eventually, subunit vaccines were developed using just a small part of an infectious agent (the part that caused the greatest immune system response). Vaccines produced through these evolving techniques have became both safer and more effective as researchers came to understand more about immunology, virology, and bacteriology — such as which small part of a virus caused a body’s immune response. This has reduced exposure of people to potentially toxic substances in an attempt to induce immunity.
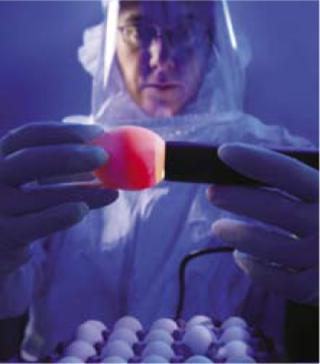
CDC microbiologist Amanda Balish demonstrates “candling” an embyonated chicken egg to determine the viability of each egg used in isolating influenza viruses. Eggs were at the heart of traditional flu virus production for most of the 20th century. ()
For years, vaccines have been administered primarily to children. So-called “childhood diseases” such as measles, mumps, and infantile paralysis (polio) have become rare in countries where children have been routinely inoculated. In recent years, the vaccine that has attracted the most public attention has to be the “flu vaccine,” a prophylactic against influenza infection. With newscasts over the past several years predicting an influenza pandemic — possibly of the latest deadly variation, the “bird flu” (H5N1) — public concern has translated into government action and focused research (and funds) on development of vaccines that might protect their recipients against the next pandemic flu. Historians remind us of the Spanish flu (a bird-flu variant of its own) that caused so many deaths almost 100 years ago. This research focus has led to some important developments in this century.
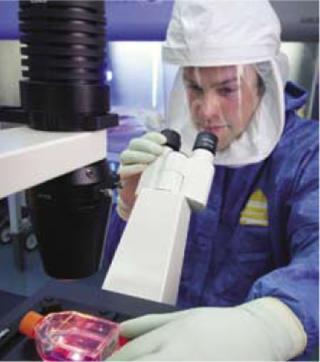
Postdoctoral fellow Neal Van Hoeven examines a culture flask of MDCK cells to look for signs of flu virus growth. Combining animal cell cultures and recombinant DNA (rDNA) technology is becoming common practice in the creation of viral vaccines. ()
Flu vaccines have long been cultured in chicken eggs. That worked well for most of the past century when, despite several serious outbreaks of newly mutated influenza viruses, a second pandemic was prevented. A cursory look at the numbers can tell even a nonmathematician that producing enough vaccine for a worldwide pandemic using egg-based technology just wouldn’t work. It would take too much time and too many eggs, and it would produce too little vaccine. So bioprocess engineers have begun looking at alternatives.
ESACT 2009 HIGHLIGHTED PRESENTATION: SESSION SIX
“Constitutive Augmentation of Heat-Shock Response in a Producer Cell for Viral Vaccines” by Ingo Jordan and Volker Sandig at ProBioGen (Berlin, Germany)
Dr. Jordon, who will be most interested in your talk, and what is your “take-home” message for them? I believe my talk touches several aspects on production of a subset of modern vaccines, namely highly attenuated vectors. For production of these vectors, specially designed cell lines are helpful. The AGE1.CR and AGE1.CR.pIX cell lines you may find mentioned in other presentations at this meeting were generated in my lab. I will focus on a specific cellular pathway called heat shock. This is a survival program for cells at the time of stress, including viral infection.
Abstract: Several viral vaccines, including highly promising vectors such as modified vaccinia Ankara (MVA), are produced on primary chicken embryo fibroblasts. Dependence on primary cells complicate production, especially in large vaccination programs. With primary cells, it is also not possible to stably express transgenes that enhance production or allow packaging of replication-deficient viral vectors. To obviate requirement for primary cells, permanent lines AGE1.CR and AGE1.CS from specific tissues of muscovy ducks were derived and further modified. We stably expressed the pIX gene of human adenovirus in the avian cells and observed increased production of unrelated MVA poxvirus.
Using modulators for heat-shock response or inflammation pathways, we observe that pIX appears to specifically activate heat shock (rather than inducing cell stress that eventually may feed into heat-shock cascades). Combining several experimental results, we speculate that the viral polymerase may benefit from the stable expression of pIX because Hsp90 is activated (possibly through Hsp70). Indeed, in the presence of pIX, proteotoxic stress has a lower impact on cells, and production of virus is less severely impaired. Thermal stability of progeny virus released by cells appears not to be affected. But comparing results from physical and functional titration of MVA, we observe an increased packaging efficiency in cells that stably express the pIX protein.
Hsp90 is described as a buffer for proteotoxic stress and a stabilizator of phenotype. pIX therefore should confer highly desirable properties to a producer cell for viral vaccines in addition to increased viral yields. However, constitutive activation of heat shock is a burden on cell proliferation and metabolism that should be addressed by medium formulation and process optimization.
Cell culture processes for producing vaccines are promising. Today, some companies are growing flu viruses (and other vaccines) in insect cell culture and some in PER.C6 human cells (licensed from Crucell NV, www.crucell.com). Some are experimenting with genetically engineered (rendered harmless) adenoviruses that present one or more flu virus proteins on their surfaces or empty virus protein cells (with no DNA or RNA), either of which should create an immune response in the body.
An even bigger step for influenza vaccine progress, however, is the idea of a subunit vaccine based on a protein common to all influenza viruses. Such a development would enable a one-size-fits-all vaccine that could protect against any influenza strain — thus eliminating the need and the guesswork of creating a new vaccine each year for the strain most likely to become widespread.
The Next Generation
Most prophylactic vaccines currently in use simply trigger the body’s own immune response and thus create antibodies that will fight off future exposures to the same toxin. Some next-generation biologics (including vaccines) are being designed to directly interact with desired cell types or receptors. Such products could turn on or turn off a specific messaging system within the cells they target, for example, or plug a “hole” that might otherwise allow a pathogen to infect. Such products would require smaller doses than current biologics, lowering production volumes and costs and increasing flexibility. Of course, recombinant vaccine technology has its challenges, including limited immune response, but companies are discovering adjuvants that can enhance the immune response.
ESACT 2009 PRESENTATIONS: NOVEL VACCINES AND VIROLOGY
Session chairs are Rod Smith and Luke O’Neill
“Toll-like Receptors: New Targets for Vaccine Adjuvants and Antiinflammatory Agents” by Luke O’Neill
“Avian Designer Cells AGE1.CR: Possible Candidates for MVA and Influenza Vaccine Production?” by Yvonne Genzel
“Constitutive Augmentation of Heat Shock Response in a Producer Cell for Viral Vaccines” by Ingo Jordon
“Efficient Production of Gene Delivery Vectors By Large-scale Transfection Technology” by Amine Kamen
“In Vitro Approaches for Improved Triple-layered Rotavirus VLP’s Quality” by Maria Candida and Maia Mellado
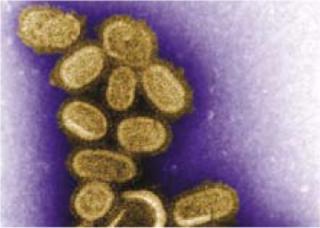
This negative-stained transmission electron micrograph shows recreated 1918 influenza virions collected from supernatants of infected MDCK cell cultures 18 hours after infection. To separate these virions, the cells were centrifuged and the viruses then fixed for negative staining. ()
Vaccines are urgently needed throughout the developing world, and cost comes into play very clearly when governments are considering vaccinating whole populations. Using attenuated viruses rather than recombinant products becomes the same kind of risk-based decision for those governments that biopharmaceutical companies are using in their production choices.
In February 2009, the World Health Organization made a deal with Schering Plough, a US drug maker, to provide improved flu vaccine technology at no cost to companies in developing countries to help them prepare for a possible pandemic. Nobilon, Schering-Plough’s human vaccine business unit, has granted WHO a nonexclusive license to develop, register, manufacture, use and sell seasonal and pandemic live, attenuated, influenza vaccines (LAIV), produced in embryonated chicken eggs. WHO will be permitted to grant a sublicense to vaccine manufacturers in developing countries working within the framework of the WHO Global Vaccine Action Plan. Vaccine manufacturers to whom a sublicense will be granted will be able to provide vaccines to the public sector of developing countries royalty-free.
ESACT 2009 Highlighted Presentation: Session Six
“Efficient Production of Gene Delivery Vectors By Large-Scale Transfection Technology” by Amine Kamen, group leader of animal cell technology at the Biotechnology Research Institute (National Research Council)
Dr. Kamen is also an adjunct professor in the department of chemical engineering at école Polytechnique de Montréal and Université Laval in Canada. He specializes in scale-up of integrated processes for adenovirus, adeno-associated virus, and retrovirus vector production. The animal cell technology group he heads is developing robust, scalable, and CGMP-compliant integrated processes for production and purification of recombinant viral vectors for gene delivery. The viral vectors of interest are adenovirus (AV), adenoassociated virus (AAV), retrovirus (RV), and baculovirus (BacMam), all of which offer potential as viral vectors for gene delivery.
The group is developing processes with SF9 insect cells (derived from the fall armyworm) for BacMam and AAV, and HEK293 cells for AdV, AAV, and RV. The research team has succeeded in developing a 100-L suspension, serum-free–medium process for large-scale expression of recombinant adenovirus vectors. In addition, it has developed a new process with a HEK293-based packaging cell line for retroviruses.
The group has also developed scalable chromatography techniques for purification of viral vectors. Ultracentrifugation is often a rate-limiting step in the scale-up process of virus concentration and purification. Alternative methods such as chromatography and ultrafiltration (cross-flow filtration) help overcome this bottleneck. Expanded-bed chromatography — a technique that combines multiple steps including separation, concentration, and capture — is being used to study the purification efficiency of viruses and plasmids. Here is Kamen’s presentation abstract:
“In previous papers we described an efficient method for large-scale transient transfection of human embryonic kidney (HEK-293) cells in suspension and serum-free medium using polyethylenimine (PEI) as a DNA carrier for accelerated production of recombinant proteins. The same approach was successfully used to generate viral vectors for gene delivery or vaccination at high yields. Recombinant adeno-associated virus, lentiviruses, and helper-dependent adenoviruses emerged in recent years as promising gene delivery vectors that may be used in the treatment of diverse human diseases. A major obstacle to broaden the use of these vectors remains the limited capacity of available production systems to provide sufficient quantities for preclinical and clinical trials. Clearly, the expansion and transfection of commonly used adherent cell lines represents a limitation to large-scale production. This presentation will describe successful strategies for production of the three vectors in stirred-tank bioreactor using suspension-growing HEK293 cells in serum-free media. The new process, based on triple or quadruple transfection using PEI as DNA transporter, allowed for scalable and controlled production that outperform standard protocols in term of safety, yield, vector function, and cost-effectiveness. The large scale transfection method appears as the most promising technology for mass-production of nonreplicating viral vectors under good manufacturing practice conditions.”
Additional Reading
1 Durocher, et al. Scalable Serum-Free Production of Recombinant Adeno-Associated Virus Type 2 By Transfection of 293 Suspension Cells. J. Virol. Methods. 144, 2007: 32–40.
2 Dormond, et al. An Efficient and Scalable Process for Helper-Dependent Adenoviral Vector Production Using Polyethylenimine-Adenofection. Biotechnol. Bioeng. 102(3) 2008: 800–810.
3 Segura et al. Production of Lentiviral Vectors By Large-Scale Transient Transfection of Suspension Cultures and Affinity Chromatography Purification. Biotechnol. Bioeng. 98, 2007: 789–799.
ESACT 2009 HIGHLIGHTED PRESENTATION: SESSION SIX
“Toll-Like Receptors: New Targets for Vaccine Adjuvants and Anti-Inflammatory Agents” by Luke O’Neil, professor of biochemistry at Trinity College in Dublin, Ireland
Toll-like receptors were first defined in detail in the late 1990s. Since then, there has been remarkable progress in our understanding of their role in the sensing of microbial products. This has led to a renaissance of interest in innate immune mechanisms among many immunologists.
For investigators interested in signal transduction, the area has proven very fruitful in the discovery of new signaling pathways and processes. MyD88 is the universal adapter for the superfamily, and its central role in inflammation, host defense, and even certain cancers has been confirmed from studies in knock-out mice.
We now have a good understanding of the major components activated by TLRs, notably the TIR domain–containing adapters that initiate signaling following recruitment to TIR domains within the TLRs themselves. Other major components include the IRAK family of protein kinases that are then recruited, and a series of ubiquitination and phosphorylation reactions that ultimately lead to the activation of transcription factors such as NF-kappaB and IRF family members.
The structural basis for signaling is still poorly understood, however, and we have no appreciation of the kinetics involved in the pathways. Additional components and regulatory crosstalk from multiple signals also continue to be discovered. Genetic variation in signaling components such as in IRAK4, Mal, and Unc93B highlight the importance of these pathways in human health and disease.
TLRs and related receptors in the NOD-like receptor family (notably Nalp3) are also of interest as targets for novel adjuvants. On the other hand, inhibiting TLRS might prove useful as an antiinflammatory strategy, wherever TLRs have been implicated in such diseases as systemic lupis erythematosis, atherosclerosis, and rheumatoid arthritis. It is hoped that the important basic findings made into TLRs now will be useful in the effort to develop novel therapies and vaccine adjuvants.
ESACT 2009 Highlighted Presentation: Session Six
“Avian Designer Cells AGE1.CR: Possible Candidates for MVA and Influenza Vaccine Production?” by Yvonne Genzel, Max Planck Institute for Dynamics of Complex Technical Systems
Can you tell me about your background? I work at the Max Planck Institute for Dynamics of Complex Technical Systems in the bioprocess engineering group of Professor Udo Reichl. In this group, I am heading the upstream processing team as senior scientist. My area of specialization is upstream processing in viral vaccine production and analytics. I have studied technical biology and since 1997 have done biotech research. Before that I specialized in biocatalysis.
Who will be most interested in your presentation? What would be your “take-home message” for them? People from the vaccine production will be interested in this information. My take home message: Avian designer cells are interesting!
Abstract: With the introduction of new designer cell lines such as AGE1.CR (ProBioGen AG) and EB66 (Vivalis), new options for viral vaccine production are at hand. When looking at influenza vaccine production with mammalian cells, either MDCK, Vero, or PER.C6 cells have been chosen as host cell lines by the industry. For modified vaccinia virus Ankara (MVA) production, only primary chicken embryo fibroblasts (CEF) have been used in the past due to host restriction toward avian cells.
Recently, ProBioGen AG has established avian cells (AGE1.CR and AGE1.CR.pIX) from duck retinas using adenovirus type 5 E1 genes and adapted them to suspension growth in serum-free medium. The CR cells were further modified by transfection for stable expression of pIX to obtain AGE1.CR.pIX cells (1, 2). Their broad spectrum in virus replication makes these new designer cell lines possible candidates for alternative production systems.
How well these cells are suited for influenza and MVA vaccine production was investigated in small-scale, stirred-tank, Wave bioreactors. General cell growth properties, metabolism, and virus production were analyzed. In addition, changes in infection parameters (virus medium, multiplicity of infection), the potential of flow cytometry measurements (cell cycle, apoptosis), and feeding strategies were considered. Results for influenza vaccine production were then compared with findings obtained for MDCK or Vero cells (3, 4).
Doubling times of 23 hours and high cell numbers (up to 7×106 cells/mL) were obtained with both cell lines. Interestingly, metabolism varied greatly between both tested cell lines and between cultivation systems. In particular, pyruvate and some amino acids seemed to be highly affected by cultivation conditions.
Both viruses were produced to high titers (3×108 pfu/mL for MVA; 3.5 log HA/100 µL for influenza virus). Particularly CR.pIX cells were highly permissive for MVA. Furthermore, various influenza virus strains (influenza A (H1N1, H3N2), influenza B) replicated well in both cell lines.
References
1 Sandig V, Jordan I. Immortalized Avian Cell Lines for Virus Production. Patent WO2005/042728 A2, 2005.
2 Jordan I, et al. An Avian Cell Line Designed for Production of Highly Attenuated Viruses. Vaccine 27(5) 2008: 748–756.
3 Genzel Y, et al. Wave Microcarrier Cultivation of MDCK Cells for Influenza Virus Production in Serum Containing and Serum-Free Media. Vaccine 24(35–36) 2006: 6074–6087.
4 Lohr V et al. New Avian Suspension Cell Lines Provide Production of Influenza Virus and MVA In Serum-Free Media: Studies on Growth, Metabolism and Virus Propagation. Submitted.
Others involved in this work were Verena Lohr, Alexander Rath, and Udo Reichl of the Max Planck Institute for Dynamics of Complex Technical Systems, and Ingo Jordan and Volker Sandig of ProBioGen.
Vaccines as Therapeutics
Today, the term vaccine may refer to a therapeutic for a chronic disease such as cancer or HIV/AIDS, rather than to a preventative injection such as flu vaccine. Therapeutic vaccines are largely produced through recombinant expression systems, which lend themselves well to platform manufacturing processes that may be used for multiple products (such as single-use technologies). These biologics may turn a system on or off or perform other such “sabotage” within the diseased cells. Other diseases that may benefit from therapeutic vaccines include hepatitis B, tuberculosis, and malaria.
One example of a therapeutic vaccine is glatiramer acetate, formerly known as Copolymer 1, which is used in patients suffering from multiple sclerosis (MS). This product, Copaxone from Teva Pharmaceuticals, resembles the myelin basic protein, which is attacked by the immune system of MS sufferers. Research into a glatiramer acetate vaccine for MS could contribute to vaccines for other autoimmune diseases including myasthenia gravis, systemic lupus, erythematosus, and rheumatoid arthritis. One major area of study for therapeutic vaccines currently is oncology.
The US FDA’s website says, “Vaccines traditionally have been used to prevent infectious diseases such as measles and the flu. But with cancer vaccines, the emphasis is on treatment, at least for now. The idea is to inject a preparation of inactivated cancer cells or proteins that are unique to cancer cells into a person who has cancer. The goal: to train the person’s immune system to recognize the living cancer cells and attack them” (1).
Regulatory agencies are studying potential issues with vaccine therapeutics, particularly the likely effects of the adjuvants necessary to induce the required response. Because adjuvants are not licensed separately from the vaccines with which they are formulated, regulatory oversight is case by case, making it difficult to create a guidance applicable to all situations. Immune responses obtained with one antigen–adjuvant combination cannot be extrapolated to another antigen — or even to the same combination given by different routes.
ESACT 2009’s novel vaccines and virology session will include presentations on “Bioprocesses for Influenza Vaccine Production,” “Constitutive Augmentation of Heat Shock Response in a Producer Cell for Viral Vaccines,” and “Efficient Production of Gene Delivery Vectors by Large-Scale Transfection Technology.” See the “Presentations” box for details.
REFERENCES
1.) Meadows, M Cancer Vaccines: Training the Immune System to Fight Cancer. FDA Consumer Mag. September–October 2004; www.fda.gov/fdac/features/2004/504_cancer.html.
You May Also Like