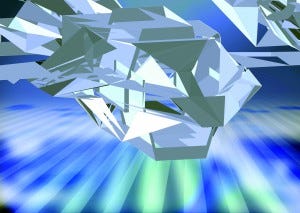
WWW.PHOTOS.COM
About 60% of all drug targets are membrane proteins. So understanding their structure and function as well as their interactions with drug candidates is critical to discovery and development of new therapeutic agents. Solubilization of these proteins is an essential precursor to in vitro studies of receptor function, structure, and activity. Purification and crystallization are important aspects. However, solubilization is complicated by the hydrophobic nature of a protein’s membrane-spanning part. Surfactant micelles and other stabilizing systems thus are used to achieve successful solubilization of membrane proteins. That use in turn necissitates a detailed characterization of protein–detergent complexes (PDCs).
Here we look at the application of tetradetector size-exclusion chromatography (SEC) for analysis of membrane proteins, particularly PDCs. A key goal of this work is to identify pure, homogeneous, stable PDCs with minimal excess detergent micelles. Such results provide a good starting point for functional assays and crystallization studies. We present an experimental protocol that can be used to optimize SEC for such applications.
Targeting Membrane Proteins
Integral membrane proteins are permanently attached to biological membranes. They constitute a full third of the human proteome and provide critical functionality to cells, both as receptors and in facilitating ion transfer. Misassembly of membrane proteins — e.g., those caused by missense mutations — can result in a broad range of diseases (1).
Detailed analysis of the membrane proteins that are responsible for specific conditions is essential to understanding of diseases and thus can guide the development of drugs that will best treat them. When it comes to assessing potential drug candidates, companies also need to analyze complexes of those molecules with membrane proteins and study their interaction mechanisms. That knowledge will help product developers successfully screen and then develop new drug candidates.
Purification and crystallization of membrane proteins is an essential precursor to their in vitro study. Successful crystallization depends on a number of factors, including protein purity and the concentration/type of detergent used. If the ratio of detergent micelles to protein molecules is too low, proteins can degrade; excess detergent can cause protein to remain in solution rather than crystallizing as required. Therefore, characterizing and optimizing the proportions of protein and detergent in a purified membrane sample provides valuable insight into the likelihood of successful crystallization. Well-characterized PDCs that are pure, homogenous, and stable — with excess micelles minimized — are optimal for both functional assays and crystallization studies.
Introducing Tetradetector SEC
SEC has applications both as a preparative and an analytical technique. It is especially valuable in production and characterization of purified PDC complexes. Used with a tetradetector array setup, SEC can measure the mass, size, homogeneity, and molecular composition of a membrane protein and its associated detergent micelle. It can also be used to determine the concentration of excess micelles. The resulting data can be used to guide crystallization studies, providing insight into characteristics of a membrane protein itself (e.g., its oligomeric state).
SEC is a two-stage process in which samples are first separated into fractions based on their hydrodynamic volumes and then analyzed using one or more detectors. Traditionally a single concentration detector — usually measuring refractive index (RI) — provides a concentration reading. With that approach, molecular weight (MW) information can be accessed only through use of relevant calibration standards. That presents a severe limitation for the analysis of complex protein conjugates such as PDCs, for which exact reference standards often are not readily available. Other modern, sophisticated systems incorporate multidetector arrays.
Research suggests that a tetradetector system comprising ultraviolet and visible absorption (UV-vis), light scattering (LS), RI, and viscometry detectors can be particularly helpful for establishing a productive protocol for PDC analysis (2). In a tetradetector array the contribution of each detector is as follows:
UV-Vis: A UV detector can be used in place of an RI detector for concentration measurements with solutes that have chromophores that absorb UV light. In PDC characterization, a Gaussian-shaped SEC optical peak is one of the simplest measures for assessing protein homogeneity (although it does not provide proof of that). This makes a UV detector useful for analyzing PDCs before crystallization and purification studies.
When UV-vis is combined with a second concentration detector, the two together can distinguish between the components of a complex such as a PDC. This allows measurement of each component’s concentration in the complex. Such a pairing of detectors therefore provides compositional analysis for protein conjugates (e.g., the amount of detergent conjugated with a membrane protein in a PDC).
RI: The concentration of dissolved sample in an eluting fraction often is measured using an RI detector, which determines concentration based on changes in a solution’s refractive index. In PDC analysis, an RI detector measures the concentration of all solutes present, thereby simultaneously monitoring the levels of PDC, free micelles, and sample contaminants. The resulting data can be used to detect excess micelles in a system, for example, thereby supporting the optimization of crystallization conditions.
Static Light Scattering (SLS): SLS detectors measure absolute MW without needing a standard that is closely similar to the sample being studied. These detectors exploit the fact that molecules illuminated by a laser beam scatter light with an intensity that is proportional to their MW, a relationship described by the Rayleigh equation (4):
As a result, MW measurements made using LS detectors do not correlate with retention time (the time the molecule remains in the column), which is usually a function of molecular size. So interactions between an analyte and the stationary phase in the SEC column do not adversely affect results. Malvern Instruments provides a more complete description of the principles of operation for LS detectors online, including a review of the advantages and limitations for different designs (3).
In PDC analysis, LS detectors allow absolute MW measurement for each of the different populations in a chromatogram. When combined with the concentration information from RI and UV detectors, that measurement can be used to derive the oligomeric state of proteins within PDCs and the amount of detergent bound with them.
Viscometry: A differential viscometer enables measurement of a molecule’s intrinsic viscosity (IV), a measure of molecular density that is related to protein MW and structure. In a multidetector array, IV and MW data can be used in combination to elucidate structural characteristics of a protein such as the extent of folding, denaturation, and broad conformational changes.
Researchers working in the department of biochemistry and biophysics at the University of San Francisco in California have studied the application of tetradetector SEC for PDC characterization in some detail and have advanced a protocol for its application (2). The experimental work presented below illustrates that approach and shows the type of information that can be generated.
A Basic Protocol For Membrane-Protein Analysis
In this protocol, a Viscotek SEC system from Malvern Instruments was used to fully characterize the properties of a PDC. It included an integrated tetradetector array with detectors connected in series as follows: UV, LS, RI, and differential viscometer. The protocol is divided into four discrete steps:
system calibration with water and column buffer
measurement of detector response factors using a freshly purified standard in column buffer
measurement of detergent dn/dc ratio (the rate of RI Change) and micelle properties using a stock detergent solution
determination of the properties of the PDC.
The separation column for this analysis was selected to match the one originally used to produce homogeneous and stable PDCs: a silica-based TSK G3000SW 0.75 × 60 cm column from Tosoh Bioscience with an additional guard column. Flow rates through the SEC system were controlled to ensure that the manufacturer’s recommended maximum column pressure drop was not exceeded and to prevent compression of the packing matrix. To minimize band broadening, we kept all connecting tubes required to link the discrete elements of this system as short as possible. Maintaining the system at an operating temperature of 4 °C provided for accurate and reproducible analysis and prevented denaturation of protein samples.
Measuring Detector Response Factors: Following equilibration of the SEC system with water and buffer, detector response factors for UV, RI, and LS detectors were measured for a purified stock solution of ovalbumin VII dissolved in column buffer. Five different column injections were performed to calculate averaged detector response factors as well as MWs, IVs, and hydrodynamic radii (Rh). Samples of 0.6 mg were injected in a 100-µL solution of 20 mM HEPES (4-(2-hydroxyethyl)-1piperazineethanesulfonic acid), an organic buffering agent; 100 mM NaCl; and 1 nM DDM (N-dodecyl-beta-d-maltoside), a detergent — to a pH of 7.4. The buffer solution ran through the SEC column at a flow rate of 0.6 mL/min.
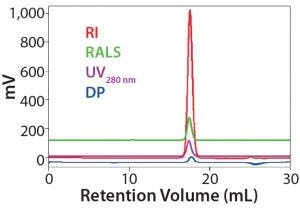
Figure 1: Data measured to determine detector calibration factors (using ovalbumin as a standard); results show that the standard is stable, with no column interactions in this buffer/column combination (1).
Results summarized in Figure 1 were highly reproducible, indicating that the standard is stable and exhibits no column interactions in this system. The resulting response factors were therefore judged to be reliable for further analysis.
Measuring Detergent dn/dc and Micelle Properties: The next step in PDC analysis is characterization of the detergent, including measurement of the dn/dc ratios for the DDM and of the associated micelles’ shapes and sizes. Injections used 20-µL to 100-µL sample volumes with masses of 0.05–1.970 mg DDM per injection. Figure 2 shows the RI data obtained, including a plot of RI area as a function of DDM mass, which we used to determine a dn/dc for the detergent of 0.130 mL/g.
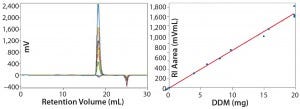
Figure 2: Data measured to determine detergent dn/dc and micelle properties; results show a good fit with micelle calculation errors <3% (1).
The second negative RI peak observed in the retention-volume plot can be attributed to a slight mismatch in HEPES and NaCl concentrations between the sample and column buffers. However, the observed fit for the dn/dc data is good, and errors for the micelle concentrations are <3%. These results indicate that the detergent micelles present are spherical, with an averaged MW of 90 kDa, and perfectly homogeneous as indicated by the Mw/Mn ratio of 1.
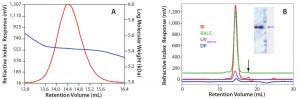
Figure 3: Data measured to determine detergent dn/dc and micelle properties; results show a good fit with micelle calculation errors <3% (2).
Measuring PDC Properties: In the final step of this protocol, we measured the properties of the PDC itself. Five column injections were performed using an injection volume of 150 µL containing 0.71 mg of PDC in column buffer. Figure 3 shows the results obtained alongside input data (B) derived from the two preceding steps of the protocol.
Comparing the RI traces for the PDC with those of DDM alone reveals that the sample contains no excess micelles based on the lack of an equivalent peak in the PDC chromatogram. Using the two concentration detectors to measure the fraction of each component in the complexes and the total mass (from LS), it is possible to calculate the mass of the protein and detergent components. Our data indicate that PDC was present as a pure heterodimer, with each dimer bound to 220 DDM molecules. Those results suggest that this PDC is pure, homogeneous, stable, and globularshaped — making it ideal for crystallization. Furthermore, they clearly illustrate how SEC can be used to measure rigorously the properties of PDCs and confirm its suitability (or lack thereof) for crystallization purposes.
Ensuring Success
The above protocol illustrates how SEC can be applied beneficially in the characterization of PDCs, and it illustrates the complexities of sequential analysis. A number of issues thus should be considered by scientists seeking to ensure that time invested in such analyses pays dividends and that a tetradetector SEC setup is fully optimized. Such issues include defining analytical goals, considering whole- and partial-peak analysis, ensuring stable baselines, selecting suitable columns and buffers, choosing calibration standards, and assessing results.
Definition of Analytical Goals: The described protocol maximizes information flow from PDC analysis but is relatively time-consuming. If the aim is crude estimation of the properties of a PDC rather than detailed characterization, then a simpler and more streamlined approach may be preferable (2). Careful consideration of your analytical aims is vital for cost-efficient application of the tetradetector SEC method.
Feasibility of Whole-Peak and Partial-Peak Analysis: Ideally, whole SEC peak analysis is preferable. But that approach is feasible only if the constituent components of a PDC produce peaks that do not overlap. If the peaks do overlap, partial-peak analysis may be acceptable. But that will produce accurate data only if the component MWs are constant across the whole peak. Results from partial-peak analysis can be highly dependent on selected integration limits and the baseline. It may be preferable to further purify such a sample to enable whole-peak analysis of it instead.
Ensuring Stability of All Baselines: A stable baseline is prerequisite for accurate SEC analysis. To maintain protein stability, low-temperature operation (~4 °C) is an additional requirement for PDCs. Good upkeep and maintenance of instrumentation are critical in reaching that goal, but state-of-the-art systems do offer features that support rigorous measurement-condition requirements. Those include high-performance degassers, temperature-controlled autosamplers, and fully integrated detector arrays that maintain samples at a closely controlled temperature throughout analysis. Such capabilities make it easier to achieve stable baselines even with hard-to-handle protein-based samples.
Selection of a Suitable SEC Column and Running Buffer: Column choice is a critical issue for all SEC analyses. Selection criteria include bead chemistry (which defines buffer and protein compatibility), particle and pore size, and column length. Silica-, sugar-, and polymer-based column matrices all are suitable for proteins, but silica columns are often the preferred choice. The latest versions boast a broad pH tolerance and excellent compatibility with a diverse range of proteins, including hydrophobic samples such as PDCs. Silica columns also are designed to minimize particulate shedding, providing a cleaner, more stable baseline for LS detection. Their separation efficiency often is good enough to enable resolution with just a single column, which constitutes an important advantage. With regard to buffer/detergent choices, the aim is to identify a system that is entirely compatible with the PDC of interest but does not bind and remains stable as a single Gaussian peak under all measurement conditions.
Choice of Standard for Detector Calibration: UV-vis, RI, and LS detectors all require calibration for sample quantification. That should be carried out using a standard with well-defined characteristics, including closely specified mass and dn/dc ratio. Furthermore, a selected calibration standard must be soluble, homogeneous, and stable in the column buffer. It must not interact with the column matrix. Ovalbumin, as used for the study described herein, is widely selected for PDC analysis.
Assess Precision and Accuracy: Repeat injections are essential to assessing the reproducibility of SEC data. Precision is especially important when parameters measured in the early stages of a protocol are used to generate results later in an analytical procedure, where an opportunity lies for errors to multiply. Again, modern instruments such as Malvern’s OMNISEC system have multiple features that are designed to improve the quality and reproducibility of data, so they can offer proven value in this regard.
Telling Results
Secure and reliable analysis of PDCs supports advancement of therapeutic agents based on their interactions with membrane proteins, which represent a fertile area of drug development. SEC is a useful technique for purification and analysis of PDCs. The protocol presented herein shows how it can be optimally applied to fully characterize PDCs and highlights the type of information that can be gathered: mass, size, shape, homogeneity, and molecular composition of a membrane PDC and its host-detergent micelle; and the concentration of excess micelles. Such data can be used to assess the likelihood of successful PDC crystallization, which is an essential aspect of in vitro studies on receptor function, structure, and activity.
References
1 Ng DP, Poulsen BE, Deber CM. Membrane Protein Misassembly in Disease. Biochim. Biophys. Acta Biomembranes 1818(4) 2012: 1115–1122; doi:10.1016/j.bbamem.2011.07.046.
2 Miercke LJ, Robbins RA, Stroud RM. Tetra Detector Analysis of Membrane Proteins. Curr. Prot. Prot. Sci. 77, August 2014: 29.10.1–29.10.30; doi:10.1002/0471140864.ps2910s77.
3 Static Light Scattering for GPC-SEC Explained. Malvern Instruments: Malvern, UK, www.malvern.com/slsexplained.
4 Characterizing Polymers: Techniques for the Measurement of Molecular Weight, Molecular Size, and Branching; Malvern Instruments: Malvern, UK, 2016; www.malvern.com/en/support/resource-center/Whitepapers/WP150420PolymerCharacterization.aspx.
Larry Miercke is a professor in the University of California, San Francisco’s department of biochemistry and biophysics. Corresponding author Stephen Ball is a product marketing manager for Malvern Instruments Ltd., Enigma Business Park, Grovewood Road, Malvern, Worcestershire, WR14 1XZ, UK; 44-1684892456, fax 44-1684-892789; www.malvern.com.