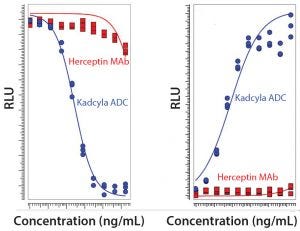
Figure 1: Comparison of unconjugated MAb and ADC in a proliferation assay with luminescence readout based on ATP release (LEFT) and a Caspase 3/7 apoptosis assay with luminescence readout to measure cytotoxicity (RIGHT) (RLU = relative luminescence units)
Seven years ago, the US Food and Drug Administration (FDA) approved the first product in a new class of biologics: antibody–drug conjugates (ADCs). The idea for these products already had been hatched a decade earlier when the promising field of antibody research — touting such molecules as “magic bullets” — had faltered, specifically against oncology-related indications. The early crop of anticancer monoclonal antibodies (MAbs) proved to have only limited efficacy, and interest in developing antibodies as therapeutic agents against cancer had faded. Two different biotechnologists simultaneously suggested that antibodies could be much more effective against cancer — if you could arm them with a chemical warhead — capable of triggering apoptosis in tumor cells (1). They hypothesized that this combination of antibody and small molecule could finally turn laboratory-produced molecules into “magic bullets”.
The idea didn’t attract investors at first, but ADCs eventually became an active area of research. There are now four FDA-approved ADCs: brentuximab vedotin for the treatment of relapsed Hodgkin lymphoma, trastuzumab emtansine for the treatment of HER2-positive metastatic breast cancer, inotuzumab ozogamicin for refractory B-cell precursor acute lymphoblastic leukemia, and gemtuzumab ozogamicin for acute myelogous leukemia (AML). Gemtuzumab ozogamicin was approved in 2000, voluntarily withdrawn by its developer for safety and efficacy reasons in 2010, and then reapproved by the FDA last year for AML patients expressing the CD33 antigen (2).
The nonprofit Antibody Society reported last year that 32 clinical trials of ADCs are ongoing, with 14 of them in phase 2 or phase 3 clinical trials (3). In 2016, the ADC market was valued at US$1.3 billion, and it is expected to reach $3.1 billion by 2022, according to the pharmaceutical analyst Allied Market Research (4). Although small molecules still command a large share of the pharmaceutical market, the industry experienced record levels of approvals for biologics in 2014– 2016. Those numbers are expected to rise even more rapidly as patents for originator MAbs expire and some biosimilars in development gain regulatory approval (5).
The Complex Structure of ADCs
ADCs are comparable with Trojan horses because they deceive tumors into allowing a cell to intake a payload of toxic material. ADCs combine the best of protein and small-molecule therapies: Antibodies are covalently joined to toxins with a chemical linker to act as drone-like weapons against tumors. Based on the binding specificity of the antibody portion of an ADC, the toxic nature of a drug’s moiety is largely masked until an ADC reaches its intended target.
Because ADCs are such complex structures, they present significant bioprocessing challenges compared with stand-alone MAbs. Both the US Food and Drug Administration (FDA) and the European Medicines Agency (EMA) treat ADCs as biologics (6, 7). But because ADCs are both drugs and biological molecules (a MAb paired with a conjugate that is typically a chemical entity such as oligonucleotide, peptide, or small molecule), analytical tests need to be appropriate for multiple biochemical and chemical species.
The three different components of ADCs (MAb, linker, and conjugate) each must be developed and manufactured under current good manufacturing practices (CGMPs). Each part (alone and in combination) must perform as desired. In addition to MAb purification and processes that keep operations free of contaminants in GMP facilities, an ADC bioprocess includes additional processes, safety measures, and analytical requirements conferred by conjugation of a highly cytotoxic component to a biologic. The linker that connects a cytotoxin payload to a MAb must be designed in such a way that it renders the payload unreactive until it is released in target cells, without affecting MAb stability. Given that most MAbs have a 72-h half-life in the circulatory system, a linker also must be stable in blood plasma for several days (8). Because cytotoxic material is so dangerous to facility workers, it must be handled in highly controlled, sterile settings by experienced personnel.
ADCs consist of a complex mixture of heterogeneous species, so they present unique bioanalytical challenges. For a small-molecule drug or a therapeutic protein, the species measured typically is the parent molecule in the latter case and the metabolite in the former. But for ADCs, it is not immediately obvious which analyte or subset of analytes will provide key information to help analysts understand a conjugate’s safety and efficacy. Moreover, limited clinical data are available for ADCs (9).
Regulatory Concerns
For companies filing an investigational new drug (IND) application for an ADC product, it is not enough to provide extensive animal toxicology and pharmacology data. They also need to offer detailed chemical, manufacturing, and controls (CMC) information about the production and stability of clinical trial material.
The quality-by-design (QbD) approach, which has been established as a guideline for pharmaceutical product development, also can be used for developing target binding assays and cytotoxicity assays in ADC development. With the QbD approach, assay development starts with the creation of an analytical target profile that defines method objectives, including critical method attributes (e.g., range, accuracy, and precision) and key method attributes (e.g., data turnaround and assay transferability) (10).
The heterogeneity of conjugation can increase the complexity of ADC samples. Therefore close attention should be paid to critical quality attributes (CQAs) that stem from the conjugation process during ADC biosimilar development. A combination of physicochemical, immunological, and biological methods is warranted to demonstrate identity, purity, concentration, and activity (potency or strength) of ADC samples.
Kadcyla (trastuzumab emtansine, T-DM1) is a good example of the complexity of ADC analysis. As a second-generation ADC, this product integrated significant enhancements over first-generation products, including a more stable noncleavable linker that reduced off-target toxicity and improved therapeutic index. Kadcyla ADC combines antitumor properties of the humanized anti–human epidermal growth factor receptor 2 (HER2) antibody trastuzumab with the chemotherapy drug emtansine DM1, a potent microtubule-disrupting agent, joined by a stable linker. This ADC is designed to shuttle emtansine DM1 into HER2-positive cancer cells without harming normal cells (11).
A number of assays can be used for characterization as well as GMP purposes to ensure both the functional nature of the ADC and its higher-order structure. Here we use Kadcyla ADC as an example to demonstrate how a joint effort coupling the functional aspects of bioassays with the structural insights from biophysical characterization ultimately can lead to a more thorough assessment of this complex biologic.
Assessing the Bioactivity of Kadcyla ADC
Production of Kadcyla ADC occurs in two major steps: addition of the linker and addition of DM1 to the linker. Trastuzumab’s mechanism of action (MoA) of trastuzumab are retained, including Fcg receptor-mediated engagement of immune effector cells that leads to antibody-dependent cellular cytotoxicity (ADCC).
In most cases, a broader range of bioassays is required to characterize a new ADC or biosimilar. The bioactivity assays chosen for lot release and stability testing are limited to one or two key assays that are reflective of the mechanism of action (MoA) of the ADC or biosimilar as well as being stability indicating.
Typically, general proliferation assays with cell viability readout and pathway-specific apoptosis assays such as Caspase 3/7 assays can demonstrate how effectively an ADC inhibits the proliferation of cancer cells and leads to programmed cell death of cancer cells. Usually, a therapeutic MAb vehicle alone is quite ineffective for inhibiting cancer cell proliferation or inducing a certain apoptotic signaling pathway. But the ADC at the same doses is capable of activating those MoAs. So both tests are important for evaluating individual lots before an ADC is released to the market or approved for use in a human clinical trial.
Other bioassays, such as ADCC and antibody-dependent cellular phagocytosis (ADCP), are used to characterize an ADC. These determine an ADC’s MoA by looking at the Fcg receptor-mediated effector function of the MAb carrying the cytotoxic payload compared with the nonlinked (“naked”) therapeutic antibody. In ADCC, target cells are destroyed by effector cells secreting molecules that can perforate a cell membrane and activate apoptosis-based cell death. In ADCP, target cells bound by therapeutic antibody are destroyed through monocyte or macrophage-mediated phagocytosis.
In addition to characterization of the bioactivity of an ADC’s MAb, the cytotoxic payload-related specific effects in vitro should be addressed. A range of different approaches might be chosen, but one typical focus is on the cell cycle arrest mediated by the cytotoxic payload. Cell cycle arrest assay (e.g., with a flow cytometric readout) reflect the MoA — when tumors cells stop dividing right before the point of mitosis. A prerequisite for that effect is the internalization of an ADC before release of its payload, which is related to the binding of MAb moiety to the receptor on the surface of the target cell. Luminescent labels and flow cytometry can be used to determine whether an ADC has delivered the cytotoxic material into a tumor cell.
Our laboratory has prepared a set of assays to evaluate the performance of Kadcyla ADC, and we presented the findings at the CASSS Bioassays meeting held earlier this year in Silver Springs, MD. Our assays show that Kadcyla ADC inhibits the proliferation of the target cells in a dose-dependent manner and that Herceptin MAb at the same concentrations had no effect. We also showed that Kadcyla activates the Caspase 3/7 pathway dose dependently, whereas Herceptin at the same concentration had nearly no effect on this pathway-specific cytotoxicity. Figure 1 shows results from the proliferation assay and the Caspase 3/7 apoptosis assay with luminescence readout.
Both approaches are suitable for use as MoA reflective assays for lot release and stability testing. And they can be validated according to ICH Q2(R1) and USP to fulfill later phase and commercial GMP requirements.
Among the characterization assays, ADCC of the ADC was reduced compared with that of trastuzumab. ADCP was enhanced in reporter-based surrogate approaches that measure how well Kadcyla ADC can activate the signaling pathways responsible for ADCC and ADCP. In general, these results vary depending on the method chosen to determine Fc-effector-function–related MoAs and might be completely different if another therapeutic antibody with a different isotype is the vehicle for the cytotoxic payload.
A third finding was that Kadcyla causes a significant cell cycle arrest at G2/M. Internalization results from in vitro models depend highly on the methods chosen to determine this prerequisite for ADC efficacy. Simple labeling of a therapeutic MAb and corresponding ADC at the Fc region is a fast and easy way to measure internalization, but steric hindrance can affect the result. So internalization of therapeutic MAbs tends to be more significant than that of the corresponding ADC at the same concentration.
The set of assays described above is suitable for characterization of upcoming biosimilars as well, but each ADC requires individual adjustment of assay parameters for the molecule.
Structural Integrity of ADCs
Another way to assess the potency of Kadcyla ADC (and biologics in general) is to use in vitro methods that assess the biophysical structure of the ADC. Biophysical assays can be used to help understand the underlying structure-based mechanism associated with a drug’s bioactivity. Biophysical tests help to address, early on, some structural differences between the innovator product and a biosimilar. Such differences should be flagged early because they could result in significantly different properties that affect efficacy, toxicity, immunogenicity, and stability.
The final folded state of a protein can be sensitive to many parameters during its expression, purification, and formulation. In fact, two protein drug substances with nearly identical primary structures can differ significantly in higher order structure because of their processing history. Biophysical techniques provide for direct measurement of a protein’s secondary, tertiary, and higher order structure — often directly in a formulation matrix. Therefore, such methods occupy an important middle ground between methods that allow for identification and characterization of the primary structure and more late-stage studies for assessment of toxicity (12, 13).
The central focus of biophysical characterization is to gain information about the structural or conformational integrity of the protein entity comprising a drug product. These issues can pertain to the nature of the folded state of a polymer chain, how individual chains can associate to form higher order structures, and the ensemble of states that might be present in a specific sample type (13). In particular, biophysical tests report on the secondary and tertiary structure of an intact molecule and on the propensity for aggregates or associated states to form.
Our laboratory recently began querying the structural aspects of the Kadcyla ADC to elucidate how the protein’s structure might influence the drug’s functional characteristics. To accomplish this, we used a number of biophysical characterization techniques for the shape, size, and aggregation state of the product.
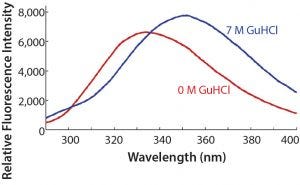
Figure 2: Intrinsic protein fluorescence spectra of Kadcyla ADC (0.5 mg/mL) in the presence and absence of 7 M GuHCl; excitation = 280 nm, emission = 290–400 nm
For example, the intrinsic fluorescence of the trastuzumab portion of the drug can be used as an efficient reporter of the folded state of the molecule. The wavelength of fluorescence emission, dominated by the tryptophan residue, will depend on the buried state of the aromatic residues. Figure 2 shows that Kadcyla ADC is sensitive to the state of denaturation, in this case induced by GuHCl, which will cause the normally buried aromatic residues to be more surface exposed.
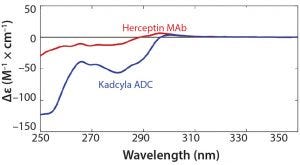
Figure 3: Near-UV CD spectra of the trastuzumab antibody (unconjugated Herceptin, 2 mg/mL) and the ADC (Kadcyla, 2 mg/mL), overlaid
Likewise, the near-UV CD spectrum of the drug product reveals a rich array of features that can be evaluated for mechanistic meaning. Figure 3 shows the near-UV CD profile of Kadcyla ADC overlaid with that of the corresponding unconjugated protein, trastuzumab. Comparing the ADC profile with that of trastuzumab shows that a number of regions stand out in the ADC as differing from that of the trastuzumab, presumably reflecting subtle differences in the local environments around aromatic residues as a result of conjugation of the small molecule. Those data, exemplified by the spectroscopic assays described above, imply that biophysical tools can be used to gain insight on structural differences that can have some bearing on the functional performance of a drug.
Characterizing the higher-order structure of a protein increases product knowledge and follows the FDA’s QbD initiative. This includes understanding batch-to-batch consistency, stability, and whether variants or aggregates can be linked to safety and efficacy. At present, assays for higher-order structure generally are not used for lot release or stability, but rather are used mainly for early and late characterization and comparability studies (14).
A Continuing Quest for Knowledge
Despite the recent clinical success of drugs such as trastuzumab emtansine (Kadcyla), brentuximab vedotin (Adcetris), and inotuzumab ozogamicin (Besponsa), the fact is that we do not yet fully understand how ADCs elicit their pharmacological effects in patients. The quest to develop more effective and less toxic ADCs continues. Improved and more predictive preclinical studies combined with clinical studies of next-generation ADCs will augment our understanding and ability to develop agents with improved pharmacological properties, reduced toxicity, and enhanced efficacy — ultimately leading to more durable clinical responses in patients (15). Biophysical assays measure protein higher-order structure and are expected to correlate well with bioassays. As measurements of in-solution conformation, biophysical assays can act as key indicators of a drug’s success. They should be understood in synchrony with functional bioassays reflecting the in vivo mechanism of action of a drug to gain a “totality of the evidence” on a developmental drug, a biosimilar, or an approved product.
As we demonstrate here, a laboratory equipped with the resources for a combination of biophysical and bioassay characterization activities is well positioned toward the goal of getting safer drugs to the market quickly. That is a win for both drug developers and patients.
Acknowledgments The authors thank Verena Sonnenberg, Sandra Bauer, Shreyas Laghate, and Michael Rivet for providing technical assistance.
References
1 Scudellari M. Tumor Snipers. The Scientist 1 November 2012; www.the-scientist.com/bio-business/tumor-snipers-40253.
2 FDA Approves Mylotarg for Treatment of Acute Myeloid Leukemia. 1 September 2017; www.fda.gov/newsevents/newsroom/pressannouncements/ucm574507.htm.
3 Melis J. Increased Clinical Pipeline of Antibody Drug Conjugates in 2016. The Antibody Society 25 January 2017.
4 Antibody Drug Conjugates Market Overview. Allied Market Research, September 2017; https://www.alliedmarketresearch.com/antibody-drug-conjugate-market.
5 Walker N. Biologics: Driving Force in Pharma. Pharma’s Almanac 5 July 2017; www.pharmasalmanac.com/articles/biologics-driving-force-in-pharma.
6 Biosimilars: Additional Questions and Answers Regarding Implementation of the Biologics Price Competition and Innovation Act of 2009, US Food and Drug Administration, May 2015; www.fda.gov/downloads/drugs/guidances/ucm273001.pdf.
7 Guideline on Production and Quality Control of Monoclonal Antibodies and Related Substances. European Medicines Agency. 5 April 2007; www.ema.europa.eu/docs/en_GB/document_library/Scientific_guideline/2009/09/WC500003073.pdf.
8 Scanlon C, Zhao J. Antibody–Drug Conjugates: Manufacturing Challenges and Trends. ADC Review J. Antibody Drug Conj. 21 March 2017; doi:10.14229/jadc.2017.21.03.001.
9 Gorovits B, et al. Bioanalysis of Antibody–Drug Conjugates: American Association of Pharmaceutical Scientists Antibody–Drug Conjugate Working Group Position Paper. Bioanalysis 5(9) 2013: 997–1006; www.future-science.com/doi/pdfplus/10.4155/bio.13.38.
10 Miller KR, Mullan A, et al. Use of Design of Experiment (DOE) for Optimization of ADC Potency Assays. Am. Pharm. Review 1 March 2017; www.americanpharmaceuticalreview.com/Featured-Articles/335152-Use-of-Design-of-Experiment-DOE-for-Optimization-of-ADC-Potency-Assays.
11 Martin C, et al. Antibody–Drug Conjugates: Design and Development for Therapy and Imaging in and Beyond Cancer. LabEx MAbImprove Industrial Workshop. 27–28 July 2017, Tours, France. mAbs 10(2) 2018: 210–221; www.ncbi.nlm.nih.gov/pmc/articles/PMC5825198/.
12 Chen L, et al. In-Depth Structural Characterization of Kadcyla (ado-trastuzumab emtansine) and Its Biosimilar Candidate. mAbs 8(7) 2016: 1210–1223; doi: 10.1080/19420862.2016.1204502.
13 Sucato C, DiPaola M. Biophysical Analysis and the Development of Follow-On Biologics. Biosimilar Devel. 11 March 2016.
14 Wei Z, Schacter E. The Role of Higher-Order Structure in Informing Biopharmaceutical Quality. BioProcess Int. 9(4) 2011: 23–27; www.bioprocessintl.com/business/cmc-forums/the-role-of-higher-order-structure-in-defining-biopharmaceutical-quality-313472.
15 Bornstein G. Antibody Drug Conjugates: Preclinical Considerations. AAPS J. 17(3) 2015: 525–534.
Corresponding author Ulrike Herbrand, PhD, Christopher Sucato, PhD, and Alvaro Jorge Amor, PhD, are scientists with Charles River’s Biologics Testing Solutions; [email protected].