Extending the Visible Residue Limit Approach to Potent Therapeutic Proteins — Part 2: Test Results and ConclusionsExtending the Visible Residue Limit Approach to Potent Therapeutic Proteins — Part 2: Test Results and Conclusions
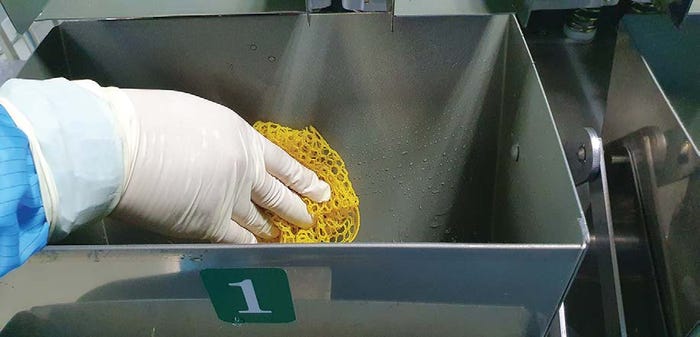
HTTPS://STOCK.ADOBE.COM
Equipment cleaning is an integral and highly regulated part of pharmaceutical manufacturing for both large- and small-molecule drugs. Before equipment release, validation engineers perform visual inspections to verify the absence of visual residue. Such inspections include examination of small parts that are cleaned in a washer.
In multiproduct biopharmaceutical facilities, product-agnostic methods such as swabbing for total organic carbon (TOC) content are used to assess product-carryover levels from one process to another (15). The TOC method’s limit of quantification (LoQ) is ~0.2 ppm. In BPI’s May 2024 issue, we began an evaluation into whether inspection based on visible residue limits (VRLs) is a viable, practical alternative for meeting cleaning requirements usually assessed by TOC.
Part 1 focused on our experimental methods, materials, and calculations. We performed a small-scale VRL study that was designed to represent worst-case conditions for visual assessment in a biomanufacturing environment. Analysts spotted precleaned 316L stainless-steel coupons with predetermined volumes of native or inactivated/degraded drug substance (DS). Those materials represented three molecules from each of three modalities: fusion proteins (FPs), monoclonal antibodies (mAbs), and Amgen’s proprietary bispecific T-cell engager (BiTE) molecules. From 1 meter away at different angles and lighting intensities, analysts inspected spotted coupons for visible residue. Our study controlled not only for observational conditions, but also for parameters such as materials of construction and solution concentrations.
Here in Part 2, we present our study results and explore the viability of VRL evaluation as an alternative to TOC swabbing for cleaning assessment.
VRL Results
Below are results of visual inspection by three analysts observing three replicates at different angles (0°, 25°, 45°) and light intensities (250, 700, and 1800 lx). When residue was visible, an analyst recorded a V for visible. If no residue was observed, NV was recorded (not visible). Our inspections also included blank (unsoiled) coupons to ensure accurate reporting of residue visibility.
Table 9 lists results for samples containing inactivated/degraded DS. Table 10 gives results for native-DS samples. Table 11 summarizes VRL scores for all product and sample types.
Table 9: From three observation angles and lighting intensities (in lux), three analysts (ANAs) performed visible residue limit (VRL) testing of coupons spotted with different concentrations of degraded drug substance from nine products representing three modalities: proprietary bispecific T-cell engager (BiTE) molecules, fusion proteins (FPs), and monoclonal antibodies (mAbs). As a control, analysts also observed blank, nonspotted coupons. (V = residue is visible to analyst; NV = residue is not visible to analyst).
Observation angle played a significant role in the visibility of soil residue. At 45°, soiling was visible to a concentration of 0.5 μg/cm2. Results were not significantly different across product modalities.
VRL Values: The visible residue limit is defined as the lowest soil concentration that all analysts can see at every angle and lighting intensity from a given distance.
Table 10: Visible residue limit (VRL) testing of coupons spotted with native drug substance; ANA = analyst, BiTE = bispecific T-cell engager molecule, FPs = fusion proteins, mAbs = monoclonal antibodies; V = residue visible to analyst, NV = residue not visible to analyst.
Degraded-Protein VRL: Our results demonstrate that although VRLs differed across tested molecules, those differences were not modality specific.
Table 11: Summary of results from visible residue limit (VRL) testing; BiTE = proprietary bispecific T-cell engager molecule, DS = drug substance, FP = fusion protein, mAb = monoclonal antibody.
Residues from BiTE molecule samples collectively were visible at concentrations of >1 µg/cm2, and residue from BiTE molecule 1 was visible at 0.5 µg/cm2. FPs collectively were visible at concentrations of >2 µg/cm2. Of note is that residues were visible to the three analysts at all angles and lighting intensities for these molecules and concentrations:
• FP 2, FP 1, and mAb 1 at 2 µg/cm2
• BiTE molecule 3, BiTE molecule 2, mAb 2, and mAb 3 at 1 µg/cm2
• BiTE molecule 1 and FP 3 at 0.5 µg/cm2.
Thus, for samples with degraded DS, residues were visible for all tested molecules at all angles and lighting conditions at concentrations of 2 µg/cm2.
Native-Protein VRL: Results from coupons spotted with native DS indicate that VRLs were molecule specific but not modality specific. For instance, residues from BiTE molecule 3 were observed at
3 µg/cm2, whereas soil from some mAbs was observed at spike concentrations as low as 0.5 µg/cm2. Recorded VRLs were
• 3 µg/cm2 for BiTE molecule 3
• 2.5 µg/cm2 for BiTE molecule 2 and mAb 2
• 2 µg/cm2 for BiTE molecule 1
• 1 µg/cm2 for FP 2 and FP 1
• 0.5 µg/cm2 for FP 3, mAb 3, and mAb 1.
VRL and TOC Values: We translated VRL results to TOC results if a coupon was swabbed and transferred to a TOC tube with 60 mL of water. We calculated maximum TOC values assuming 100% recovery from surfaces. Calculations accounted for residue containing active pharmaceutical ingredient (API) only (TOCAPI) and for residue containing all product components (TOCAll).
For degraded proteins, the maximum theoretical TOC concentration for a swab sample is 0.46 ppm (Table 12). That calculation assumes that the residue comes from API only, representing worst-case conditions. However, such residues can include any or all of a drug product’s components, not just the API. Adjusted for actual recovery levels, TOC values will be significantly less than 0.46 ppm. Considering total carbon content of all product components, the maximum theoretical TOC value is 0.44 ppm.
Table 12: Correlation of visible residue limit (VRL) results to total organic carbon (TOC)
values for coupons spotted with degraded drug substance (DS, swabbed area = 25.8 cm2,
sample volume = 60 mL); the Max TOCAPI value is the maximum total organic carbon
content, assuming that the residue contains only active pharmaceutical ingredient (API).
Thus, that value represents a worst-case condition. The Max TOCAll value assumes that
the residue contains all DS components. (ppm C = parts per million, calculated).
For native-DS samples, the maximum theoretical concentration for a swab sample is 0.685 ppm (Table 13). Adjusting for actual recovery, TOC will be significantly less than that value, which represents the worst-case scenario that residue contains API only. When accounting for all product components, the maximum TOC value is 0.570 ppm.
Table 13: Correlation of visible residue limit (VRL) results to total organic carbon (TOC)
values for coupons spotted with native drug substance (DS, swabbed area = 25.8 cm2,
sample volume = 60 mL); the Max TOCAPI value is the maximum total organic carbon
content, assuming that the residue contains only active pharmaceutical ingredient (API).
Thus, that value represents a worst-case condition. The Max TOCAll value assumes that
the residue contains all DS components. (ppm C = parts per million, calculated).
Conclusions
Three observers inspected stainless-steel coupons spotted with inactivated/degraded DS at three different angles and light intensities from 1 meter away. Both lighting intensity and observation angle significantly influenced the visibility of soiling. Lighting of 1800 lx prevented analysts from seeing residue on the coupons. In addition, a viewing angle of 0° greatly reduced the likelihood of observing soiling. Slight angling in combination with lighting of <1800 lx should produce ideal conditions for soil visibility. Product modality did not appear to have a significant effect on soiling visibility. Keep in mind that during visual inspection in cleanability studies, coupons are observed from a close distance at multiple angles and under a fixed light intensity. In such conditions, residues from sample concentrations of >0.5 μg/cm2 are visible.
Our results were similar for coupons spotted with native DS. Both lighting intensity and observation angle played a significant role in whether soiling was visible at very low concentrations. Product modality did not appear to influence residue visibility.
Based on our results, we conclude that VRL is a viable alternative to TOC-swab acceptance limits of <0.7 ppm for native protein and <0.5 ppm for degraded protein. Because proteins usually degrade during cleaning processes, VRLs can be used in place of swab acceptance limits as low as 0.5 ppm. Those conclusions are supported by the consistency of our results, which we generated under controlled conditions and for multiple protein products of different modalities. We also assert that, for degraded proteins, a VRL of 2 μg/cm2 would be product independent and applicable for all therapeutic proteins of the three tested modalities. Likewise, a native-protein VRL of 3 ug/cm2 would be product independent and applicable to all proteins of the three modalities assessed. Our results also are consistent with those presented in other evaluations of VRLs for small-molecule APIs, supporting the generalizability of the VRL concept to a broad range of drugs (9–14).
References
9 Desai P, Walsh A. Validation of Visual Inspection as an Analytical Method for Cleaning Validation. Pharm. Online 11 September 2017; https://www.pharmaceuticalonline.com/doc/validation-of-visual-inspection-as-an-analytical-method-for-cleaning-validation-0001.
10 Ovais M. Statistically Justifiable Visible Residue Limits. Pharm. Technol. 34(3) 2010; https://www.pharmtech.com/view/statistically-justifiable-visible-residue-limits.
11 Fletcher DI. Determination of Surface Visible Residue Limits on Pharmaceutical Plant Equipment. Pharm. Technol. 37(2) 2013: 48–51; https://www.pharmtech.com/view/determination-surface-visible-residue-limits-pharmaceutical-plant-equipment.
12 Forsyth RJ, Van Nostrand V. Visible Residue Limit for Cleaning Validation and Its Potential Application to a Pharmaceutical Research Facility. Pharm. Technol. 28(10) 2004: 58–72; https://www.pharmtech.com/view/application-visible-residue-limit-cleaning-validation-richard-j-forsyth-and-vincent-van-nostrand.
13 Forsyth RJ, Van Nostrand V. Using Visible Residue Limits for Introducing New Compounds into a Pharmaceutical Research Facility. Pharm. Technol. 29(4) 2005: 134–140; https://www.pharmtech.com/view/using-visible-residue-limits-introducing-new-compounds-pharmaceutical-research-facility.
14 Fourman GL, Mullen MV. Determining Cleaning Validation Acceptance Limits for Pharmaceutical Manufacturing Operations. Pharm. Technol. 17(4) 1993: 54–60; https://www.pharmtech.com/view/setting-cleaning-validation-acceptance-limits-topical-formulations.
15 Kouda R, et al. Extending the Visible Residue Limit Approach to Potent Therapeutic Proteins, Part 1: Materials and Methods. BioProcess Int. 22(5) 2024: 30–35; https://www.bioprocessintl.com/qa-qc/extending-the-visible-residue-limit-approach-to-potent-therapeutic-proteins-part-1-materials-and-methods.
Corresponding author Ram Kouda, PhD, is senior principal scientist in process development at Amgen, 1 Amgen Center Drive, Thousand Oaks, CA 91320; [email protected]. Syeda Tabassum is a scientist in process development, Travis Saks-Rudd is a CW-MCS manufacturing associate, and David Dolan, PhD, is scientific associate director of global environmental health and safety (EH&S) at Amgen. Kurt Schnittgrund is a consultant and validation engineer at Azzur Group.
You May Also Like