Bioprocess manufacturing systems have incorporated single-use/disposable components for more than 50 years and have demonstrated well-defined process benefits from their use (1,2,3,4,5,6). The environmental impact of single-use technologies, however, has been a major focus of attention only in recent years. This evolving interest has been driven by many factors including concerns over environmental change, emissions, and energy supplies; rapidly increasing costs and restrictions on waste disposal; greater recognition of the role of disposables in bioprocessing; and availability of a wider range of disposable options.
For those reasons, today’s process designers and users must be in a position to assess and provide data on the environmental impact and sustainability of their manufacturing systems. This task can be very difficult and challenging, especially for complex multistage processes, whether involving traditional stainless steel equipment or disposables. Local factors such as restrictions on waste disposal or water resources, energy costs, and so on will also play a vital role in any assessment. Fortunately, there are many sources of information (7,8,9,10) that can facilitate an environmental assessment including guidelines from industrial organizations (e.g., BioProcess Systems Alliance, BPSA); regulatory guidelines and directives; data from suppliers and manufacturers; and published articles, reviews, and case studies.
This study focuses on power consumption from fossil-fuel sources, such as this coal-burning plant in Seal Beach, California.
Development of single-use technologies has focused not only on scale-up of well-established components (such as flexible biocontainers, filters, and connectors), but also on the development of disposables for a broader range of unit operations. For example, options are now available for bioreactors, stirred compounding vessels, product transfer systems, capture and polishing chromatography, tangential-flow concentration and diafiltration, virus removal, and many other unit operations. Some of these are at an early stage of development, and their application may be restricted in some processes by factors such as scale-up limitations, incompatibility of process fluids, or extreme operating conditions. Nevertheless, wherever use of a disposable option is feasible, it is important to assess the environmental impact of the technology so that new processes — whether in disposable, traditional stainless steel, or hybrid systems — can be designed with a better understanding of their environmental consequences.
Environmental impact can be assessed in a number of ways: e.g., disposal methods for liquid and solid waste, carbon footprint of an overall process, and a full life-cycle analysis for materials and components. In later publications, we will present other aspects of environmental impact such as waste disposal methods, waste management guidelines for specific components, and cost analyses. Energy consumption was the measure of environmental impact for our study, which forms a part of a course in environmental natural sciences at the Swiss Federal Institute of Technology in Zürich, Switzerland. We had two objectives:
To calculate the energy consumption for individual components in a model multistage bioprocessing system
To compare the total energy consumption of the same process using a traditional stainless steel system and a disposable system.
Model Systems
The model systems shown in Figures 1 and 2 are based on unit operations typically found in monoclonal antibody (or other recombinant protein) production processes at the 1,000-L scale. Design of these model systems involved discussions with a major biopharmaceutical manufacturer and several manufacturing plant visits. Some unit operations (designated by a pink-checked background in these figures) are common to both systems, so they excluded from the comparative study.
ENERGY CONSUMPTION CALCULATIONS
Materials: Table 1 summarizes data used as a basis for energy calculations for materials. Most plastic components are shown as polypropylene even though they may contain other polymers. This generalization for calculations was possible because energy conversion values for different plastics are very similar, except for polyamide. From the weight of a component and the number of cycles in its life-span, it was possible to calculate the material weight per cycle (Table 2). These figures include plastic packaging materials for both systems. Conversion of material weight to energy consumption used the data listed in Table 3.
Sterilization: For the stainless-steel-based process, energy consumption for steam sterilization was based on a steam generator output of 500 kW/h and an energy value of 8 MJ/kWh derived from electricity generated by a mix of fossil fuel and hydroelectric power. For the disposable process, energy consumption for gamma irradiation at 25 kGy for the 28 components was based on 90 components/pallet at three pallets per hour and an output of 38 kWh.
Cleaning: Calculations were based on using equal quantities of distilled pyrogen-free water, 1 M sodium hydroxide and 1 M phosphoric acid per cleaning cycle. Table 4 shows the related energy conversion calculations.
Physical Constants: Table 5 shows physical constants used in the calculations.
Figure 1: Stainless steel cleanable system
Table 1: Materials data used for components of model systems
Table 2: Weights of raw materials in model systems
Table 3: Energy conversion values for materials
Table 4: Energy conversion values for cleaning
Table 5: Physical constants
Stainless Steel Cleanable System: For this system (Figure 1), the hardware is a combination of stainless steel and some reusable, cleanable plastic components. For descriptive purposes, however, we describe it as the stainless steel system as well as cleanable, reusable, and multiuse. The process uses standard cleaning chemicals such as 1 M sodium hydroxide and 1 M phosphoric acid; pyrogen-free water for product compounding, buffers, cleaning agents, and rinsing: and 130 °C steam for sterilizing appropriate parts of the system.
Disposable/Single-Use System: Figure 2 shows the disposable system model. There are several differences between it and the multiuse system. Stainless steel buffer and storage tanks are replaced by flexible biocontainers in stainless steel totes. All vent filters are eliminated. Liquid filters in stainless steel housings are replaced by disposable capsule filters. Bioreactor exhaust-gas filters in stainless steel housings are replaced by disposable capsule filters. And capture and ion-exchange polishing chromatography columns and resins are replaced by disposable membrane absorber capsules. Some unit operations involve hybrid systems with a combination of single-use and cleanable components: e.g., capture chromatography and concentration/diafiltration. All disposable components are presterilized with gamma irradiation at 25 kGy.
Figure 2: Model disposable single-use system
Calculations and Comparison
Three key functions were analyzed for energy consumption: sterilization, cleaning, and materials. Energy consumption for other aspects of this process was excluded, including transport and installation costs (which are both relatively insignificant compared with the key functions). Some technical data for energy calculations was supplied by the biopharmaceutical manufacturer involved in discussions on the model systems. Other information came from reference documents (11) and personal communications. The “Energy Consumption” box explains the basis for calculations and constants used.
System Sterilization: Certain information and assumptions were necessary to establish a basis for sterilization calculations. For the stainless steel system, energy originates from electricity (from fossil fuels and hydroelectric power in this study). About 100 L of water is required to provide 130 °C steam for 30 minutes. And pipework and filters are sterilized together with the storage tanks, except for two filters that are steam-sterilized separately.
Components of the disposable system are presterilized with >25 kGy gamma irradiation.
Comparative calculations (Figure 3) show that the stainless steel system consumes 2,000 MJ of energy, a relatively high value due to the high cost of steam generation and the heat capacity of stainless steel hardware. By comparison, the disposable system consumes only 32 MJ because of the low energy consumption of gamma irradiation.
Figure 3: Energy consumption for
sterilization
Cleaning: Certain information and assumptions were necessary to established a basis for cleaning calculations. For the stainless steel system, cleaning agents are sodium hydroxide and phosphoric acid at 1 M concentration, and distilled pyrogen-free water is used. For the disposable system, all components were single-use with no cleaning.
Comparative calculations (Figure 4) show that the stainless steel system consumes 4,929 MJ of energy, reflecting the high energy demand for production of pyrogen-free distilled water (WFI). By comparison, the elimination of cleaning for disposable systems reduces this energy consumption to zero. Energy use for cleaning a stainless steel system may be lower if purified water can be produced by lower-energy methods. Also, investment in highly specialized designs of cleaning equipment may reduce requirements for cleaning agents and rinse water without compromising cleaning efficacy.
Figure 4: Energy consumption for cleaning
Materials: Certain information and assumptions were needed to establish a basis for materials calculations. For the stainless steel system, liquid filters are changed after every batch, and air filter cartridges are changed after every 20 batches. Stainless steel hardware has a lifetime of 600 production batches. System pipework is 10 m in total. And packaging materials are included in the energy calculations. All components in the disposable system are used only once. Ten meters of flexible tubing are used, and packaging materials are again included in the energy calculations. Polypropylene was assumed for all plastic components except the polyamide flexible tubing. Comparative calculations (Figure 5) show that the energy consumption for materials in the stainless steel system is 1,090 MJ. The disposable system gives a greater value of 4,124 MJ due to higher materials use. Where suitable facilities are available, it may be possible to recover some energy consumed by disposables through heat recovery (cogeneration) from incineration of the solid waste or conversion into fuel by pyrolysis.
Figure 5: Energy consumption for materials
Total Energy Consumption: If the calculated energy consumption values for sterilization, cleaning, and materials are combined (Figure 6), the total value for the stainless steel system is 8,018 MJ, and for the disposable system it is 4,156 MJ.
Figure 6: Total energy consumption
Discussion and Conclusions
Energy consumption is an important and measurable parameter for assessment of environmental impact for process systems. The results of this study show that if only one parameter had been chosen for analysis, such as materials or cleaning, a very distorted assessment of energy consumption would be obtained. Assessing three specific process operations together — namely sterilization, cleaning, and materials — provides a more balanced assessment. The disposable process in this model gave total energy consumption values that were about half those of the traditional system. Additional parameters such as installation and transport could change the ratio to some extent. They could not be included because precise data were unavailable at the time of the study, although rough estimates indicate that the balance of energy consumption would remain significantly lower for disposables if those parameters were taken into account. Different model system designs, operating procedures, and utilities may give slightly different though not major changes to the overall energy balance.
We hope that the methods and calculations used here will be of value to others preparing assessments on their own systems. This study may also be of value to those wishing to carry out a wider and more comprehensive environmental assessment in which other parameters are measured (e.g., waste disposal methods, full life-cycle analysis for materials, and environmental sustainability). Some of those aspects will be covered in later publications on environmental impact.
For suppliers of single-use process components and systems, there is already an expectation to provide system designers and users with freely available information on disposable components: e.g., validation data, qualification protocols, and certifications as well as systems design and engineering support (12,13,14). As environmental issues become increasingly important in manufacturing industries, suppliers may be expected to support their products also with comprehensive environment-related data such as that presented in this study and to demonstrate a responsible approach to this important topic.
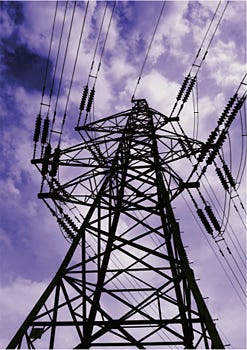
LYNNE LANCASTER (WWW.SXC.HU)
REFERENCES
1.) Langer, E. 2008.5th Annual Report and Survey of Biopharmaceutical Manufacturing Capacity and Production, BioPlan Associates Inc., Rockville.
2.) Sinclair, A, and M. Monge. 2005. Concept Facility Based on Single-Use Systems: Part 2. BioProcess Int. 3:S51-S55.
3.) Fuller, M, and H. Pora. 2008. Introducing Disposable Systems into Biomanufacturing: A CMO Case Study. BioProcess Int. 6:30-36.
4.) Pora, H. June 2006. The Case for Disposable Manufacturing Equipment to Accelerate Vaccine Development. BioPharm Int..
5.) Haughney, H, and J. Hutchinson. 2004. Single-Use Systems Reduce Production Timelines. Gen. Eng. News 24.
6.) Liu, P. 2005. Strategies for Optimizing Today’s Increasing Disposable Processing Environments. BioProcess Int. 3:S10-S15.
7.) Wells, B. 2007. Guide to Disposal of Single-Use Bioprocess Systems. BioProcess Int. 5:S24-S27.
8.) 2000. Directive 2000/76/EC of the European Parliament and of the Council of 4 December 2000 on the Incineration of Waste. Off. J. European Communities http://eur-lex.europa.eu/LexUriServ/LexUriServ.do?uri=OJ:L:2000:332:0091:0111:EN:PD:91-111.
9.) Guide for Industrial Waste Management, US Environmental Protection Agency.
10.) Article 16 of and Annex II to Council Directive 1999/31/EC 1999. Criteria and Procedures for the Acceptance of Waste at Landfills, European Union.
11.) Atkins, P, and J. De Paula. 2006.Atkins’ Physical Chemistry, Oxford University Press, Oxford.
12.) BioProcess Systems Alliance (BPSA) Disposal Subcommittee 2008. Guide to Disposal of Single-Use Bioprocess Systems. BioProcess Int. 6:S24-S27.
13.) Mach, CJ, and D. Riedman. 2008. Reducing Microbial Contamination Risk in Biotherapeutic Manufacturing: Validation of Sterile Connections. BioProcess Int. 6:20-26.
14.) Ding, W, and J. Martin. 2008. Implementing Single-Use Technology in Biopharmaceutical Manufacturing: An Approach to Extractables/Leachables Studies, Part One — Connections and Filters. BioProcess Int. 6:34-42.
15.) Botterill, M, and B. Rawlings. 2008. Applying Good Engineering Practices to the Design of Single-Use Systems. BioProcess Int. 6:18-25.