Subunit vaccines stimulate immune cells by delivering selected components of a pathogen of interest rather than the entire pathogen. Elicio Therapeutics is developing subunit vaccines that target a recipient’s lymph node to elicit a robust immune response. The vaccines are based on the company’s amphiphile (AMP) platform (Figure 1). The platform-based drug candidates are targeting cancer and COVID-19. Their development would allow the vaccine to be stored without the need for ultracold and cold storage.
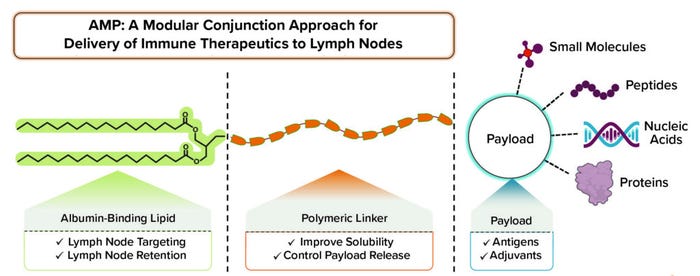
Figure 1: The amphiphile platform enables lymph node delivery of validated therapeutics with modular application.
Below, we discuss the development of Elicio Therapeutics’s vaccine candidates and AMP platform with Peter DeMuth, PhD (chief scientific officer) and Chris Haqq, MD, PhD (executive vice president, head of R&D and chief medical officer at Elicio Therapeutics).
Vaccine Development
What drove Elicio to work with subunit vaccines?
Haqq: It’s a bit of a misnomer to call our vaccines traditional subunit vaccines. Vaccines generally stay at the site of injection. Elicio’s AMP technology delivers target molecules into a recipient’s lymph nodes. That capability can improve vaccine potency significantly compared with what has been possible thus far. We leverage the key advantages of subunit vaccines, including their long-term safety, but we don’t need to make an entire pathogen protein. We believe that our AMP technology could reach a new level of effectiveness and safety by combining vaccine agents and trafficking them to the lymph nodes. We also can link different payloads onto our technology’s AMP group. We’ve done that already with peptides, proteins, and nucleic acids. In principle, we could attach other biologics or small-molecule drugs to send them to lymph nodes as well.
We are taking a differentiated approach with our platform. If you compare it with that for mRNA vaccines, for example, you’ll find that mRNA vaccines can be made quickly but require frozen storage. Our AMP-based vaccines rely on traditional chemistry for their synthesis and are easy to scale. We are testing their potential for room-temperature formulations so that they wouldn’t need cold storage.
What processes go into the chemical synthesis you mentioned, and how are those processes scaled up?
Haqq: The technologies that we use are quite amenable to scale-up. Our lead program, ELI-002, includes peptides and nucleic acids. Those are made on large-scale synthesizers based on column synthesis, then attached chemically to a lymph-node–targeting AMP group. A helpful consideration here is that we can use technologies that are already in place for scaling up production of peptides and nucleic acids. Looking ahead, we’ve calculated that if, for example, we reached large-scale synthesis for nucleic acids (such as for our AMP–CpG adjuvant) and kept a single manufacturing suite in continuous operation for our ELI-005 candidate against SARS-CoV-2, then we could produce enough drug substance to manufacture a billion doses per year.
What therapeutic areas are you targeting?
Haqq: ELI-002 is our leading clinical-stage program in a dose-escalation trial evaluating its safety and efficacy as a treatment for patients with [Kirsten rat sarcoma viral oncogene homologue] KRAS-driven tumors who have minimal residual tumor cells following surgical removal of a tumor. That study is active now across the United States in major oncology centers, including Memorial Sloan Kettering Cancer Center (New York, NY), Massachusetts General Hospital (Boston, MA), the University of Texas MD Anderson Cancer Center (Austin, TX), Washington University Medical Campus (St. Louis, MO), the Henry Ford Health System (Detroit, MI), and City of Hope National Medical Center (Duarte, CA).
KRAS mutations are among the most common mutations in cancer. They are found in 25% of all solid tumors that get diagnosed every year. More than 90% of pancreatic cancer patients have a mutation in the KRAS gene. About half of colorectal cancer patients have such a mutation, as do about 30% of non–small-cell lung-cancer patients. Our dose-escalation study will include multiple tumor types with KRAS mutations.
One interesting feature of our ELI-002 candidate is that the stretch of amino acids that we target within the RAS [rat sarcoma virus] protein is identical across three separate genes: the KRAS gene and the closely related NRAS [neuroblastoma RAS] and HRAS [Harvey rat sarcoma virus] genes. HRAS mutations are quite common in bladder cancer, and NRAS is common in AML [acute myeloid leukemia] and other tumors. Although our initial clinical trial is not studying patients whose cancers have such mutations, in theory our drug could offer benefits to those patients, as well.
Some emerging cancer treatments such as cell and gene therapies have tight development timelines. How do your subunit vaccines’ timelines compare?
DeMuth: Subunit vaccines generally have a favorable storage potential. For example, we’ve shown that our COVID-19 vaccine candidate can be stored at room temperature for multiple days or at refrigerated temperatures for multiple weeks. There might be an opportunity to improve on that even more with our AMP approach. Some features with the technology will provide additional measures of stability to agents that are modified relative to their conventional counterparts. In that context, we believe that the AMP platform has the potential to provide an off-the-shelf approach.
It’s interesting that you mentioned cell therapies because we commonly think that we are trying to deliver all the benefits of a cell therapy but use the vaccine to transfer the “manufacturing” to inside a patient. We can think of lymph nodes almost as a small factory. They can produce cells that you otherwise would be giving as a therapy after manufacturing them in a laboratory. That entails all the challenges that you just mentioned. So the vaccination approach has a lot of benefits to offer in terms of supply chain, patient access, and timing.
Haqq: I’m an oncologist by training. When I graduated in 1996 from medical school, I think we had only one or two curative regimens for patients with widely disseminated diseases. But those came by combining several cytotoxics with different mechanisms. I think what we’ll see in the immune therapy space will be just the same. We’re going to see agents such as amphiphiles become key pillars because they can compensate readily for the defectiveness of some other immune therapies.
You can look at highly successful drugs such as PD-1 [programmed cell death protein 1] checkpoint inhibitors and notice that they are not approved for indications such as colon and pancreatic cancer, which have a lot of unmet need. That is because those tumors lack the T cells within those checkpoint inhibitors. A drug such as ELI-002 primes T cells in a lymph node. So we are interested in advancing combination therapies as ELI-002 progresses in our pipeline.
The great thing about immune therapeutics compared with cytotoxics is the durability of responses. In our preclinical models, even upon rechallenge, many of the mice that are initially in complete remission remain so indefinitely as long as we can keep them alive. That’s a promising result that could be particularly beneficial for patients.
What are some milestones that you’ve reached with the ELI-002 and ELI-005 candidates, and what do you consider to be future development challenges?
Haqq: In addition to being in the clinic with our ELI-002 candidate, we are taking steps toward the next level of synthesis. We are making both AMP-CpG and AMP peptides at relatively small scale for initial development. We have good pilot data to conduct process development and are performing formal [good manufacturing practice] GMP engineering runs to scale that up. We’ll continue with those activities until we reach the largest-scale synthesizer so that we can conduct such work broadly around the world. In parallel, we’re working on stable liquid formulations, and we’d like to develop lyophilized formats.
DeMuth: We have achieved preclinical milestones for those programs, with studies in both mice and nonhuman primates. We’ve been able to validate not only the efficacy of our lead candidates, but also a mechanism that underlies all AMP technology, which is the lymph-node–targeting ability of those agents. Our studies serve as a proof-of-concept for our platform and for individual products that are in development. We are using simple standard of practice for manufacturing and scale-up, following established paradigms, and moving up in scale.
In terms of clinical practice, especially as it relates to the ELI-005 COVID-19 therapy program, evolution of a virus always is a concern. How do you develop a vaccine that will be relevant for today and for variants that emerge a year and a half from now? We’ve observed that getting agents into lymph nodes with the AMP approach provides a response that is extensively broad in its ability to recognize variants that did not exist at the point when we were developing ELI-005. I think that’s in part because we are educating the immune response in the lymph nodes, where there is a unique ability to recognize an antigen and develop persistent, broad immune specificity for a pathogen. That is something that we must keep our eye on as we go from drug design to development.
AMP Platform Technology
Why is the platform called an amphiphile? Is it because it’s hydrophilic and lipophilic?
DeMuth: Yes, exactly. When we were designing the AMP platform, we wanted a structure that would allow for noncovalent physical association with proteins that carry agents through the lymphatics. One of those proteins is albumin, one function of which is to carry fatty acids around the body. The structure that we designed includes a lipid, which is a natural ligand for albumin, and we took into account the way that our amphiphiles bind onto albumin to be carried from an injection site to a lymph node. We also discovered that to optimize that interaction, we needed a hydrophilic block — usually a polymer — to promote pharmaceutical properties such as solubility. The resulting structure ended up having lipophilic and hydrophilic properties.
What makes that structure a platform in design, and how do can it be used in future therapies?
Haqq: We performed separate GMP manufacturing of AMP-CpG because that agonizes the toll-like receptor 9 (TLR9) and dendritic cells and revs up costimulatory pathways such as CD28 and 4-1BB. That’s what sends a “danger signal” and gets T cells to engage. Then, we vary the antigen from the KRAS protein to the SARS-CoV-2 antigens to any other pathogen of interest, depending on use. By having that plug-and-play aspect to our platform and the separate GMP manufacture, we’re ready to go.
With a future clinical candidate, we wouldn’t need to do a large amount of GMP scale-up because we already have the key adjuvant piece, the AMP-CpG. So if we observe good safety results in our existing clinical trials, that should set the stage for a large number of combinations.
DeMuth: AMP platform development has been focused on making it something that can be applied readily across different targets. We can evaluate new targets and then develop a new product within a calendar quarter or two. The process is building off what we know as a field already in terms of designing good agents. The focus, however, is getting those agents where they can be most effective. Our ELI-005 candidate is a good example. Although our company was focused on oncology before the pandemic, we are now investigating how the AMP platform could be used to target COVID-19 and other infectious diseases.
How does the production of AMPs compare with that of vaccine-delivery platforms such as lipid nanoparticles (LNPs)?
DeMuth: Taking mRNA vaccines as an example, the key difference is that LNP manufacturing involves producing precursor materials. Those are added to a complex mixture and then undergo a complicated set of steps to formulate a self-assembled particle with mRNA in the middle and the lipid corona on the outside. We’re not doing that complicated formulation. We are making agents and purifying them to achieve our specifications. They are formulated together in aqueous buffer conditions. Because those agents are amphiphilic, they will self-assemble, in some cases into higher-order structures such as micelles. The mechanism of action in the body does not depend on that drug form.
Those interactions are not long lived. They are easily broken apart in vivo by different endogenous proteins or just diluted out. That is common for micelles. Once the interactions are broken, that’s when the “magic” begins and amphiphiles can bind onto albumin. We’re not relying on the particle structure at all for drug efficacy in vivo, just on a by-product of the physical and chemical properties of the agents and how they exist in aqueous-formulated drug-product conditions. I think that’s the main difference with AMPs and other lipid particles. Whereas mRNA-encapsulating LNPs rely on an intact particle form to achieve their mechanism of entering cells and drive expression of the mRNA to produce the protein (which then becomes the active agent of the drug), our AMP molecule is less complex and has a simplified mechanism of action.
How does the platform’s immunogenicity compare with other vaccine types?
DeMuth: As a class, subunit vaccines are attractive because they have a history of both safety and an ability to generate protective antibody responses. But as Chris mentioned, subunit vaccines generally are designed to remain at the injection site, which is a significant limitation because most immune responses that we want to generate through vaccination will be developed, supported, and coordinated at the lymph nodes. AMP molecules can deliver vaccine agents more effectively to lymph nodes than can other modalities. That provides those agents with an opportunity to engage actively and optimally with immune cells that also are present in the lymph nodes (Figure 2).
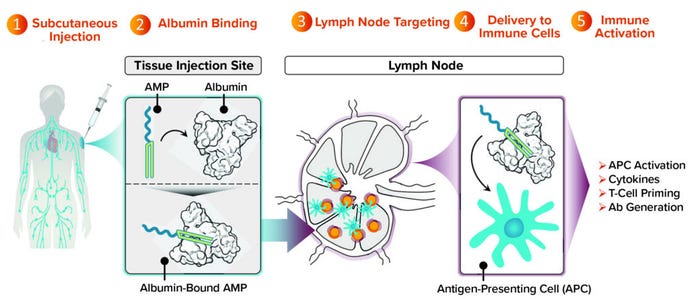
Figure 2: Targeting lymph nodes with AMP technology to orchestrate immunity.
We’ve completed studies in mice and nonhuman primates showing that an AMP approach not only maintains the strength of antibody response that historically subunit vaccines have been good at producing, but also induces T-cell responses. That result is considerably improved compared with what is possible with conventional subunit vaccines. Many such vaccines are designed to stay at the injection site and produce undetectable levels of T cells in preclinical models. With AMP-directed vaccines, we find T cells circulating in the bloodstream and observe them in tissues that are important to the progression of a target disease (e.g., lung or spleen tissue).
For our ELI-005 candidate against COVID-19, our studies show that the vaccine stimulated production of many long-lived T cells that could recognize different variants of SARS-CoV-2. Achieving that result has been a challenge for some COVID-19 vaccines. The facts that our AMP platform can generate good neutralizing responses from antibodies along with T-cell responses that are durable and can broadly recognize variants of disease as they emerge are promising developments.
Scalability and Logistics
Are there logistical challenges or problems with delivery and administration?
Haqq: From a clinical perspective, not really. One key thing that we have been finding in our clinical studies are the many genomic sequencing technologies. Upon diagnosis, many cancer patients learn that they have mutations in their RAS genes. Currently, some small-molecule therapies such as the Lumakras (sotorasib) product from Amgen have been commercialized, but those cover only one variant, the G12C. Our product candidate can target seven different mutations. One of the more striking parts of our clinical trial is that many patients know already that they have an indication for which experimental therapy is available. They seek out our agent because of its broad spectrum.
DeMuth: That also applies to our COVID-19 vaccine program, ELI-005. We talked already about subunit vaccines having some benefits regarding the supply chain. Unlike mRNA or some other platforms, they don’t require ultracold storage. Thus, our therapies can be distributed in different areas of the world, especially in low- or middle-income countries (LMICs).
Administration for our products is quite similar to other vaccines that most people have received as an injection into the arm or leg. After that, the AMP system is designed to target agents directly into the lymphatics. There’s no special dosing or administration technique that we need to practice. Doses can be administered by a nurse or trained healthcare worker in the middle of the desert, a clinic, or local pharmacy, depending on the indication. For a future prophylactic vaccination, that could fit easily into the model that’s been established for mass vaccination campaigns across the world. Similarly, oncology vaccine administration could take place in out-patient or hospital settings.
Haqq: We hope that our candidates will become prophylactic vaccines in the future. I’m the child of a cancer patient who had a G12 mutation. So I have a personal stake in our work. If a vaccine is safe in cancer patients, then you can imagine that everyone who has a relative who has developed a mass tumor could get that vaccine as a preventative. Because such a large proportion of cancer patients have those mutations, there is the potential that we could eliminate almost half of the cases with a prophylactic vaccine. The vaccine would be effective with all who are RAS positive, for example. That also applies to those who have pancreatic cancer, 90% of whom have a KRAS mutation. Although it would be great to use a product in a preventative setting, those achievements are still a bit down the road for us. We are not yet conducting clinical trials in such a setting.
You talked about the different payloads that can be included alongside an AMP molecule. Is there a limit on the type of payload that can be carried?
DeMuth: Our AMP platform was built to provide flexibility. We’re fortunate to be living in a time when immunotherapy as a field and vaccine science as a field have defined an exciting repertoire of different therapeutic agents, all of which are designed to influence the immune response and drive it to treat cancer and infectious diseases. The over-arching drawback of many such therapeutic agents is that they are not doing as well as they could be because they’re not getting to lymph nodes. The platform we designed applies to different classes of payloads. We’ve reduced it to practice for large classes such as peptides, oligonucleotides, small molecules, and even small proteins, which together encompass a broad range of therapeutic agents.
We believe, however, that such agents are leaving much activity behind if they are unable to get to lymph nodes effectively. So our strategy is to “correct” the reasons that those agents aren’t doing as well as they could be by getting them efficiently to lymph nodes and giving them the opportunity to influence the immune system in ways that they have not been able to in the past.
Haqq: That also is exciting from a clinical point of view. We know that there is a group of pathogens that are controlled by antibody responses. We’ve seen a good example of that with COVID-19. But other pathogens such as tuberculosis are controlled by T-cell responses. Today, we don’t have effective vaccines for those. We see “night and day” changes in which traditional vaccination doesn’t elicit detectable T-cell responses. Yet with the lymph node engagement, we’re seeing huge numbers of T cells emerge that have the appropriate response specificity to the pathogen. That could open up therapies for fighting several pathogens in the infectious disease space.
Our company is focused on oncology right now. But there could be huge opportunities on the infectious-disease side because many pathogens are known to be controlled through T-cell response. The flip side of that is autoimmune diseases, for which we can use our method to deliver cargo to lymph nodes. For example, we could deliver something that shuts down the immune response and something that turns it on. By doing so, you could treat many prevalent autoimmune diseases such as lupus or multiple sclerosis. That’s something that we also think about.
We’ve talked a bit about scalability and capacity. Is there anything you’d like to add regarding how your processes compare with those for other vaccines?
DeMuth: By getting agents into lymph nodes more effectively, we can reduce the dose that is needed to produce the same or better response in the immune system. That’s because we are getting more of the agent directly to where the immune response lives. We don’t have to manufacture as much material because we don’t have to dose as much. The same amount can be used to dose more people, potentially. In a pandemic or for infectious diseases, that capability has value because you don’t have to produce huge amounts of drug to treat the world’s population.
Haqq: Manufacturing costs for some drugs such as cell therapies are high. Using the human body as a “bioreactor” can be advantageous because when amphiphiles bring those transferred cells into the node, they can be expanded inside a patient. You could dose much smaller numbers of cells but give them together with an amphiphile that would promote “manufacturing” inside patients.
Our data are showing that when tumors in mice are cleared, that is partly the result of antigen spreading. The orchestration of those responses in lymph nodes ends up not being confined to just the part that was targeted; it expands to other antigens. Durability has been a key point to think about. The fact that we see that spreading advantage coming into play when a lymph node revs up suggests a potency that I don’t think has been observed in other drug products yet. Recruiting a patient’s own T-cell responses against a tumor to become much stronger alongside a targeted antigen could mean all the difference between having a cure and not.
Maribel Rios is managing editor of BioProcess International; [email protected]. Brian Gazaille is associate editor of BioProcess International; [email protected].