Effective Cryopreservation and Recovery of Human Regulatory T CellsEffective Cryopreservation and Recovery of Human Regulatory T Cells
The list of conditions being targeted by cell therapies is rapidly growing, but commercializing cells for widespread medical use will require standardized laboratory practices. Development processes must be adapted specifically for cell-based drug products. Regulatory T-cell therapy represents a promising new frontier in the immunotherapy of autoimmune disorders, especially for patients who have been refractory to available treatments. Because of intrinsic fragility, cell therapy products can be highly sensitive to variations in manufacturing procedures. Standardization of drug-product cryopreservation and storage steps are thus key to ensuring consistent clinical trial results.
Regulatory T (Treg) cells represent a cell population that can modulate immune responses and inhibit inflammatory processes in vivo. Treg cells maintain homeostasis of the immune system, preventing its unwanted activation against self-antigens (autoimmunity) or those that are normally tolerated (e.g., dietary antigens, inhaled antigens, and bacterial flora antigens).
Tregs modulate immune response by controlling the activation and proinflammatory properties of other immune cells, including conventional CD4+ and CD8+ T cells, natural killer cells, B cells, and antigen presenting cells such as dendritic cells and monocytes. Multiple mechanisms of Treg immunosuppression have been elucidated. They are mediated through direct cell–cell contact, secretion of soluble antiinflammatory cytokines, secretion of cytotoxic granules, and modulation of the inflammatory milieu by degradation of extracellular adenosine triphosphate (ATP). That arsenal of mechanisms-of-action gives researchers multiple cellular and molecular targets, making Tregs an appealing strategy for treating patients with chronic inflammatory diseases. This is especially true for those who are refractory to conventional therapy, which involves single-target and systemic approaches using immunosuppressors and therapeutic monoclonal antibodies (MAbs).
Tregs in the Clinic
For those reasons, Tregs are being brought to clinical trials for treating a number of diseases linked to autoimmune disorders: type 1 diabetes, graft versus host disease (GVHD), and Crohn’s disease. Crohn’s disease is a chronic inflammatory disorder of the gastrointestinal tract, with which patients often experience courses of relapse and remission. Conventional treatment involves the use of steroids, immunosuppressors, and anti–tumor-necrosis-factor (TNF)-α agents, which bring about remission and help heal the intestinal mucosa. However, about a third of the patients treated are refractory to anti-TNF treatment, leaving with them with no further recourse.
Recently Desreumaux et al. completed a 12-week, open-label, multicenter, single-injection, escalating-dose, phase 1–2a clinical study involving 20 patients with refractory Crohn’s disease to test a Treg cell therapy named Ovasave (1). This study used autologous, antigen-specific, type 1 Tregs (Ag-Treg) that target the diet antigen ovalbumin (Ova-Treg). The Ovasave manufacturing process involves isolation of peripheral blood mononuclear cells (PBMCs), ex vivo selection and expansion of Ova-Treg, cryopreservation of the resulting product in a controlled-rate freezer, and subsequent cell thawing immediately before infusion. Results of this study show a good tolerability of Ovasave injection and clinical response in 75% of patients treated with the best apparent dose. Therefore, Ovasave product development will continue with a phase 2b clinical study to confirm the beneficial effects of this product for refractory Crohn’s disease. Process developments will aim to ensure a robust and consistent manufacturing process for this autologous product.
Challenges in Cell Therapy Trials
Cell-based therapy production has intrinsic variability that is difficult if not virtually impossible to eliminate. Inconsistent sample handing leads to difficulties in accurately judging the clinical efficacy of cell-based therapies. As new products move through clinical trials, it will be critical for the successful completion of clinical trials for companies to establish readily scalable protocols that ensure consistency and minimize human error.
To maximize reproducibility in the clinic and achieve statistically meaningful results, it will be necessary to identify steps in which variation can be eliminated. To produce cell therapy products, companies need to focus on sample handling and minimize study variability. Sample storage temperatures affect cell viability and recovery, so it is a critical aspect of sample handling on which to focus during product development. Most notably, improper temperature control can introduce experimental variability during cryopreservation processes. Successful cryopreservation depends on the proper rate of temperature decrease, followed by long-term storage in liquid nitrogen. When cryopreservation steps are applied in cell-based clinical studies, variabilities in that process can lead to severe consequences to their clinical outcomes.
For the Ovasave cell therapy trial, cryopreservation of the drug product is critical because a single blood sample is used to make doses for several years of patient treatment. In addition, one key in-process control (IPC) of the manufacturing process requires freezing a patient’s peripheral blood mononuclear cells. These freezing steps require that cells be frozen at a controlled rate of –1 °C/min.
For early clinical trial phases, freezing was performed using a controlled-rate freezer. Although that was effective, such freezers have several significant drawbacks: They are expensive, they have a large footprint, and their use is specific to cell freezing. Controlled-rate freezers also can be difficult to operate and prone to malfunction; they require careful location of a minimum of two thermocouples in the freezing chamber and on the samples to be cooled. If those sensors are not connected appropriately, the control system will no longer receive accurate data and respond with incorrect inputs of liquid nitrogen and subsequent cooling (2).
Here we describe and test a cost-effective, scalable alternative to controlled-rate freezers, demonstrating its successful adoption in a phase 2b clinical cell therapy trial. The CoolCell cell-freezing device has been designed to freeze cells reproducibly, uniformly, and consistently at a rate of –1 °C/min in a –80 °C freezer.
Materials and Methods
Clinical-grade production of ova-Tregs is detailed in a previous publication by Desreumaux et al. (1). Briefly, peripheral-blood mononuclear cells were collected from healthy donors and isolated by Ficoll gradient centrifugation. To expand ova-specific T cells, PBMCs were cultured in the presence of ovalbumin and supplemented with interleukin 2 and 4 (IL-2, IL-4). After seven days, T cells were cloned and then selected based on an ovalbumin-specific IL-10 production.
For handling and freezing, cell aliquots were transferred to closed-system 2-mL glass ampules from Aseptic Technologies. The freezing media contains LFB 4% human serum albumin and either 10% dimethyl sulfoxide (DMSO) for PBMCs or Cryostor-10 from Biolife Solutions for Ag-Treg cells. We used the same media for experiments with both the controlled-rate freezer and the CoolCell freezing device from BioCision. PBMCs were frozen at a concentration of 3 × 107 cells/mL, and Ova-Treg cells were frozen at varying dosage. We analyzed cell viability with propidium iodide staining and subsequent flow cytometry and absolute cell count with flow cytometry (using a MACSQuant system and Propidium iodide solution from Miltenyi Biotec in Cologne, Germany).
To use a CoolCell freezing device in a European Union good manufacturing practice (GMP) manufacturing facility cleanroom, its polyethylene exterior was sprayed with two surface-cleaning ag
ents/disinfectants: first a solution of Surfanios/Hexanios quaternary ammonium salts (Laboratoires Anios), and then Anioxyspray solution of hydrogen peroxide and alcohol (Laboratoires Anios). We left those surface disinfectants in contact with the device for 15 minutes before gently wiping it with 70% ethyl alcohol and rubbing it dry. After the cleaning procedure, the particle emission profile was verified using a Climet Instruments CI-3100 particle counter and the microbial effectiveness of the cleaning procedures using Biomerieux Count- Tact gelose plates. We tested the particle-release performance three times on three different CoolCell freezing devices (for a total of nine measurements).
Results
PBMCs and Ova-Tregs were cryopreserved in either a CoolCell freezing device or a controlled-rate freezer programmed to freeze at –1 °C/ min. The former is a passive freezing device made of highly insulative closed-cell polyethylene (Figure 1) coupled with a solid-alloy thermal core to fine-tune and balance its freezing profile to –1 °C/min. The CoolCell device ensures a uniform freezing rate in all vials by a radial distribution pattern that provides them with an identical rate of thermal energy loss in a low-temperature environment. Once loaded, the full device is transferred into a –80 °C freezer for passive controlled-rate cell freezing.
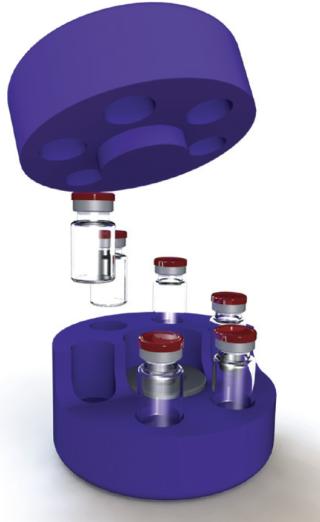
Figure 1: ()
We used the CoolCell freezing device in back-to-back experiments with only a 5–10 minute wait period between runs. It requires no isopropanol or other fluids. Its simple design is durable and provides for a nearly indefinite product life cycle without apparent change in performance.
Five consecutively measured profiles yielded nearly identical patterns of freezing (Figure 2). The device provided a freezing rate of –1 °C/minute for each sample just as a programmable freezer would. Based on those observations, TxCell tested the suitability of CoolCell freezing devices for use in the Ovasave Phase 2b cell therapy clinical study.
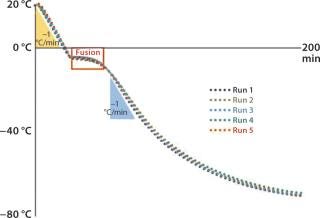
Figure 2: ()
We assessed postthaw cell viability of healthy donor PBMCs frozen in a CoolCell freezing device and compared the results with PBMCs frozen in a controlled-rate freezer. Prefreezing viability was consistently >95%. Cells were frozen and maintained at –80 °C for 12 hours and then stored at –150 °C for five days. After thawing them, we evaluated cell viability with flow cytometry and observed no significant difference between PBMCs frozen using either method (Figure 3). We found the biological function of PBMCs frozen using the CoolCell device to be unaltered. They retained the capacity to behave as antigen-presenting cells in vitro (data not shown).
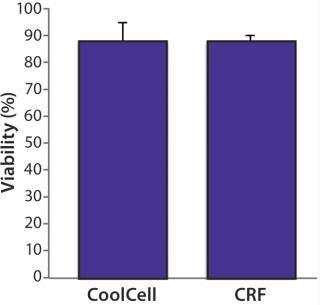
Figure 3: ()
FDA guidelines for cell therapies require cell viability to be >70% for the release of cell therapy product lots (3). To test our application of the CoolCell freezing device with clinical cell therapy products, we compared postthaw viability and yield of Ova- Tregs frozen using a CoolCell device and a controlled-rate freezer. In these experiments, prefreezing viability was consistently >90%, and we observed no significant difference in viability or yield between cells frozen using either method (Figure 4). Postthaw cell viability was 91.7 ± 3.7% from the CoolCell device and 91.7 ± 4.0% from the controlled-rate freezer. Cell yield was 83 ± 13% from the CoolCell device and 88.8 ± 10.7% from the controlled-rate freezer.
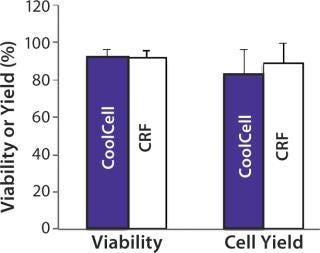
Figure 4: ()
Ex vivo cell processing and subsequent cryopreservation must be performed in approved cleanrooms for cell therapy products. GMPs require adherence to standard cleaning procedures for all equipment used in cell processing. To determine whether the CoolCell freezing device would be suitable for cleanroom use, we conducted particle-release performance tests, in which CoolCell freezing devices were subjected to standard cleanroom equipment cleaning procedures. Release of particles ≥0.5 µm and 5.0 µm were evaluated against EU GMP annex 1 specifications (4). Notably, the maximal limit of particle release permitted in an active class B cleanroom is 352,000 for particles ≥0.5 µm and 2,900 for particles ≥5.0 µm. Repeated tests showed that particle release was significantly lower than the maximum allowed (Figure 5). Our studies also show that standard repeated equipment cleaning and decontamination is effective to contain microbial contamination to a level suitable for a class B cleanroom.
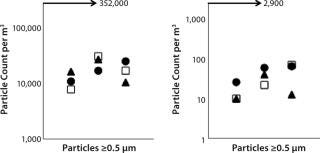
=”font-size:71.429%;”>Figure 5: ()
Discussion
Standardizing the collection of human biological samples either before or after ex vivo manipulation is a significant challenge in clinical medicine. Variability in sample/ product handling can lead to differential patient outcomes (5). For cell therapy production, an adequately controlled freezing of the final drug product before long-term storage is a prerequisite to ensuring consistent integrity and therapeutic effect. This is particularly relevant in the context of chronic treatments. One approach to mitigating risks linked to sample freezing is to explicitly document all sample-handling protocols and minimize the opportunity for error by using simple devices.
The CoolCell freezing device is a suitable alternative to a controlled-rate freezer for development of cell therapy products. We tested the device because it offers several advantages over controlled-rate freezers for clinical applications. It is cost-effective and requires no specialized equipment beyond a –80 °C freezer, which is standard equipment in laboratory settings. It requires no maintenance, further reducing associated cost. Additionally, it is straightforward to use, which minimizes the chance for errors in sample handling.
Our data clearly demonstrate that incorporation of the CoolCell device into a cell therapy trial provides the same freezing outcome as controlled-rate freezers. Postthaw cell viability after freezing with the CoolCell device easily exceeds the minimum viability required to meet FDA cell therapy requirements (3), so the cells are deemed suitable for patient infusion. They also retain their function after cryopreservation. Furthermore, we show that the device is suitable for use in active clean rooms. It seamlessly integrates into the workflow of cell therapy product manufacturing with no special training required.
Based on these results, TxCell is adopting the CoolCell freezing device as the method of freezing antigen-specific regulatory cell (Ag-Tregs)– based product for the Ovasave Phase 2b trial. In early preliminary trials, TxCell used electronic controlled-rate freezers to manage the cell-freezing temperature. While providing the same freezing outcome as a controlled-rate freezer, the new device is much easier to use because of its portability and lack of needed maintenance. In addition, for future scale-up and scale-out of Ovasave manufacturing, the CoolCell device can provide an easy and cost-effective process transfer solution for the freezing step.
All cell therapy clinical trials are conducted following GMPs, but ensuring consistency of batches produced with cells from different donors is still a challenge. Attention is growing in the field toward variability in sample handling that confounds trial results and thus is not in the best interest of patients. Specifically, the efficacy of Ag-Tregs can change without proper and reproducible handling methods. Standardization of preanalytical sample handling, cryopreservation, and storage are key to ensuring consistent trial results (6).
Looking forward, we believe that careful scrutiny of ex-vivo protocols with the aim of minimizing variability between lots and between cell processing sites will pay large dividends in the success of cell therapy trials. Carefully controlling every aspect of how cells are handled, including the temperature at which they are stored and the process by which they are frozen, is the best way to ensure that the full potential of a clinical trial can be realized. Standardization of cell therapy manufacturing is a stepwise approach and should allow the field to deliver efficient, competitive, and approved new therapeutic options for patients with still-unmet medical needs.
About the Author
Author Details
Arnaud Foussat is vice president of research and new product development, Rodney Rietze was senior direct of process development, Eric Pottier is a qualified person and vice president of supply chain, all at TxCell SA, Les Cardoulines HT1, Allée de la Nertière, 06560 Valbonne-Sophia Antipolis, France. Brian Schryver is vice president of product development, corresponding author Maria L. Thompson is vice president of scientific affairs, and Rolf O. Ehrhardt is chief executive officer at BioCision, LLC, 12 East Sir Francis Drake Boulevard, Suite B, Larkspur, CA 94939; [email protected]
REFERENCES
1.) Desreumaux, P. 2012. Safety and Efficacy of Antigen-Specific Regulatory T-Cell Therapy for Patients with Refractory Crohn’s Disease. Gastroenterol. 143:1207-1217.e2.
2.) Shu, Z. 2010. Development of a Reliable, Low-Cost, Controlled Cooling Rate Instrument for the Cryopreservation of Hematopoietic Stem Cells. Cytotherapy 12:161-169.
3.) CBER 2008. Guidance for FDA Reviewers and Sponsors: Content and Review of Chemistry, Manufacturing, and Control (CMC) Information for Human Somatic Cell Therapy Investigational New Drug Applications (INDs), US Food and Drug Administration, Rockville.
4.) 2009. EU Guidelines to Good Manufacturing Practice: Medicinal Products for Human and Veterinary Use — Annex 1: Manufacture of Sterile Medicinal Product. EudraLex 4.
5.) Andrew, D. 2012. Variability in Blood- Based Amyloid-Beta Assays: The Need for Consensus on Pre-Analytical Processing. J. Alzheimer’s Dis. 30:323-336.
6.) Golab, K. 2013. Challenges in Cryopreservation of Regulatory T cells (Tregs) for Clinical Therapeutic Applications. Int. Immunopharmacol. 16:371-375.
You May Also Like