Fetal bovine serum (FBS) was — and in many cases still is — the supplement of choice to maintain the viability of mammalian cells in culture. However, there are considerable limitations to its use. In the early days of cell culture, the issues surrounding serum were mainly its variable performance and the potential to contaminate cultures with fungi, viruses, and bacteria. Early attempts to produce a serum-free medium (SFM) were academic exercises that usually relied on the use of purified or semipurified extracts of serum (or other biological material) to replace the growth factors and hormones normally present in it (which were necessary to maintain cell viability). Although such early “serum-free media” were more defined, they still contained animal-derived agents and were in many cases very expensive to use.
In recent years, the scale of mammalian cell culture manufacture has increased to cope with the large-volume demands of the next generation of protein-based therapeutics. In the biopharmaceutical industry, the issues surrounding serum-use have not diminished — and in fact, other reasons to limit or eliminate its use have been highlighted. These include reducing and/or eliminating the potential to transmit latent viruses and prions (e.g., bovine spongiform encephalopathy, BSE) to a final drug product and the increasing costs of serum. From a biomanufacturer’s perspective, the increasing investment required to develop biotherapeutics has made keeping manufacturing costs as low as possible and ensuring a consistent and robust upstream phase into important factors for a viable manufacturing process. Furthermore, the introduction of large quantities of protein into a bioreactor typically adds unnecessary downstream processing costs to achieve an acceptable level of product purity (1).
PRODUCT FOCUS: PRODUCTS OF ANIMAL CELL CULTURE
PROCESS FOCUS: PRODUCTION, PROCESS DEVELOPMENT
WHO SHOULD READ: QA/QC, PROCESS DESIGNERS, MANUFACTURING
KEYWORDS: FETAL BOVINE SERUM, SERUM-FREE, MAMMALIAN CELL CULTURE, SUPPLEMENTS, RISK
LEVEL: INTERMEDIATE
Due to safety and process constraints with the use of serum, development of an effective serum-free medium for culturing mammalian cells became a major focus for media development scientists. To regain the growth-promoting effects of serum, SFM was often supplemented with animal-derived components such as protein hydrolysates of bovine origin and other animal-derived proteins such as growth factors and albumin (2,3,4).
Alongside issues of product safety and the constant pressure for lower cost of goods sold (COGS), the past 10 years have seen media development directed toward protein-free media (PFM). As understanding of nutritional requirements for specific production clones has increased, the development of chemically defined and protein-free (CD–PFM) media has become possible. Although good results have been obtained for individual manufacturing processes, no single CD–PFM has demonstrated satisfactory productivity results across a broad range of cell types and culture conditions. Adaptation of each production clone to specialized CD–PFM for optimal cell line stability and biological performance can be laborious and time consuming, which negatively affects process timelines.
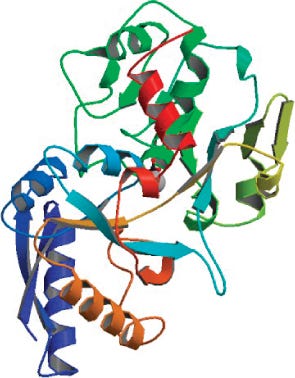
Figure 1:
Protein Database image of transferrin protein (WWW.PDB.ORG)
Reducing Risks
Many regulatory authorities have issued guidance documents regulating the use of serum or any animal-derived materials to minimize the risk of contamination of the final product (5). If animal-derived supplements cannot be eliminated, guidelines allow biomanufacturers to assess and document their associated risk based on the type and origin (biological and geographical) of each supplement. Ethical considerations are also being raised regarding the manner in which FBS is collected, which places pressure on the academic and industrial markets to seek alternatives to the use of serum (6).
The ability to develop a safe and effective serum-free (SF) production medium is a major focus for media development scientists. To date, it has been extremely difficult to develop a universal medium capable of supporting the growth and recombinant expression in different cell lines (e.g., CHO, NS0, BHK21, and HEK293) to levels that are currently expected by the industry. Successes have been limited to particular clones, and optimization typically requires a large amount of time and resources to achieve. Often the process needs to be repeated for each subsequent production clone.
The use of animal-derived supplements that were once acceptable (e.g., albumin, transferrin, fetuin, insulin, and cholesterol) now requires strict sourcing requirements for their use. Of these, insulin has been available in a recombinant form for many years and is a common bioactive protein supplement used in SFM. When a recombinant form is unavailable, many manufacturers have turned to chemically based alternatives: chelated iron in place of transferrin, pluronic F68, cyclodextrins, and even hydrolysates in place of albumin.
In the past 10 years, the level of understanding regarding nutritional and environmental requirements of mammalian cells in large-scale production cultures has dramatically increased. This has allowed some manufacturers to successfully develop chemically defined, production media for particular cell clones. PFM offers some major benefits (cost, safety, ease of purification); however, most mammalian-expressed biotherapeutics on the market or in clinical development contain a growth factor or bioactive supplement in their production media (e.g., insulin, IGF-I, or other recombinant growth factors). That is because PFM are generally less robust than are more complex media, and the time to adapt cell clones to PF conditions can be extensive.
The Cellular Environment
Mammalian cell lines originate from an in vivo environment rich in nutrients that help cells survive and perform their normal functions. Such cells are also exposed to a very protein-rich environment and thus are under the influence of many bioactive proteins. This is in stark contrast with the environment inside a bioreactor — particularly so when suboptimal culture media are used. In vivo, various proteins act either as hormones to promote secretion, as mitogens to induce proliferation, or as survival factors in response to noxious external stimuli.
Recently, recombinant forms of the earlier animal-derived media supplements (transferrin and albumin) have become available. Together with insulin and IGFs, they make up some key bioactive components found in sera. Although other growth factors are commercially available for use (e.g., FGF2, PDGF, EGF), their cost has often been a prohibitive factor often leading to the decision not to use them in the cost-sensitive biopharmaceutical industry.
Nonetheless, an increasing range of cost-effective, recombinant proteins offers media development scientists an expanded array of supplements to help them optimize production media for popular mammalian cell lines. Moreover, we may discover that cells are better able to use the nutrients present in the resulting media. It is well known that although Fe2+ and Fe3+ can enter cells, it is the presence of transferrin (Tf) and interaction with its receptor that targets Fe3+ to the correct organelle for efficient use by a cell (7). This may account for the better performance of cells often observed when Tf is present (8). Insulin is well known to influence cellular metabolism, and feeding strategies may have a greater impact when insulin is present than when it is not (9).
Not only do bioactive proteins have individual effects on cells, but they often work in concert. Some resulting actions are quite complex and can lead to very dramatic changes in cell proliferation and biosynthetic performance. It has been shown that fibroblasts respond to a combination of EGF and IGF stimulation that is positively synergistic for growth (10). Sunstrom et al. showed that coexpression of both IGF-I and transferrin was necessary to achieve suitable growth of CHO cells in culture (11). Recently a recombinant form of human transferrin was developed — CellPrime rTransferrin AF (rTf) by scientists at Novozymes Biopharma — that was found to perform as well as the native molecule for a number of cell types (12,13).
Figure 1: ()
EXPERIMENTAL REPORT
BY TONY SIMULA
We recently investigated the influence of combining rTf with LongR3 IGF-I recombinant factor on the performance of two commercially relevant cell lines: two CHO DukxB11 clones, each producing a human MAb, and a Sp2/0 hybridoma clone secreting a mouse MAb. The cells were tested in both 96-well microplate and suspension culture studies. Growth in microplates was determined by measuring the metabolic reduction of MTS tetrazolium dye (in the CHO cells) or by CyQuant DNA fluorescence (in the Sp2/0 cells). Growth in suspension cultures was determined directly using a Guava cell analyzer. IgG productivity was determined by analytical HPLC using a protein A column (for the mouse MAb) or by ELISA (for the human MAb).
The influence of each factor was initially tested separately in a 96-well plate model and then in combination based on well-established beneficial concentrations of both supplements in basal media. Initial studies with CHO Clone A were extensive, looking at two time points (day 6 and day 10) for growth and productivity. Both factors positively influenced the growth of that clone (Figure 1). An even greater effect was observed when they were combined in the basal medium, with the combined influence more obvious at day 6. Differing patterns of growth between days 6 and 10 can be accounted for by the assay method: Wells are typically inoculated at the study’s initiation but not fed again during the experiment. So although differences in growth at day 6 are noticeable, by day 10 the growth has essentially reached a plateau. The combined factors appeared to have an even more dramatic effect on the productivity of this clone (Figure 2). The pattern was the same at both days 6 and 10, which would indicate that although growth appeared to reach a plateau at day 10, the cells’ ability to secrete MAb was not compromised and was positively influenced by the factors.
To confirm those results, a second CHO clone was also tested in the same type of assay, looking at only a single concentration for each factor and at one time-point, day 6 (Figure 3). The relative effects of the individual factors were qualitatively similar to those observed with the first clone (Figure 2). And in this instance the combination of factors again had a significant synergistic impact on growth (p < 0.05). Similarly, productivity of the second CHO clone was also greatly improved by use of the combined factors and was much greater (p < 0.05) than the sum of their individual effects (Figure 4).
To determine the extent of this effect on other cell types using a similar format, we assessed growth and productivity of the Sp2/0 clone over 6 and 10 days after treatment with LongR3 IGF-I factor, rTF, or both. In this instance the former had no or a negative effect on cell growth, and by contrast rTf significantly improved growth (Figure 5). The positive effect of rTf is not surprising because Sp2/0 cells often require larger quantities of Fe3+ in their medium, and addition of rTf should improve intracellular Fe3+ transport (12). When the two factors were supplied in combination, growth was significantly (p < 0.05) improved over the effect observed for rTf alone. The benefit of combining these factors was even more evident when productivity of the Sp2/0 clone was measured (Figure 6), and the pattern was similar on both day 6 and day 10. Preliminary studies with the same clone grown in suspension cultures showed the same patterns of activity when both factors were used in combination (data not shown).
Discussion: Of the more important defined, bioactive recombinant media supplements, only insulin and IGF-related molecules have been available to a suitable quality standard and in commercially sustainable quantities. With the recent launch of CellPrime rTransferrin AF factor, there is now another animal-free factor that can be considered when developing a production medium. It performs as well as native human transferrin and outperforms the commonly used chelated forms of Fe3+ (12,13). The results described above are consistent with a study by Sunstrom et al., who demonstrated that only when both proteins were coexpressed in a wild-type CHO clone was any growth benefit observed (11). In that study, their influence on productivity was not investigated. It is well documented that insulin and IGF-I can activate similar intracellular pathways (16). Recent data from our laboratory have confirmed that results seen here can be repeated for a combination of IGF-I or insulin with rTf but not with inorganic iron salts (as shown in the “Synergy” box).
Figure 1: ()
Figure 2: ()
Figure 3: ()
Figure 4: ()
Figure 4: ()
Figure 5: ()
Defined Supplements for Robust Media Design
The scarcity of protein in CD–PFM media tends to make their performance more sensitive to changes in culture conditions such as nutrient limitation, stability, and sensitivity to shear stress. Native proteins are derived from animal blood fractions (albumin, transferrin, fetuin) or tissues (insulin, IGF-I, EGF) were once common serum-free media supplements. Although beneficial in cell culture, use of some such supplements (e.g., albumin and transferrin) has declined over the past decade because recombinant, animal-free forms have been unavailable (14,15). Recombinant forms of those proteins (and others) have been developed recently. Such defined, animal-free proteins show comparable biological activity and productivity performance to their animal-derived counterparts.
For many years, growth-factor supplements have been an essential requirement for optimal cell growth in culture. Specifically engineered for supplementation of cell culture media, LongR3 IGF-I animal-free recombinant growth factor acts directly on the IGF receptor (IGF-IR) for maximal activation of key mitogenic and antiapoptotic pathways to enhance proliferation and survival of mammalian cells (16,17). This growth factor demonstrates performance that is equivalent or superior to insulin in supporting cell growth and productivity (Figure 1). Moreover, it offers a distinct biological advantage because of its reduced binding affinity for inhibitory binding proteins produced by most mammalian cells (a result of an amino acid substitution at position three of the IGF-I molecule). Independent studies have shown this product to be a superior growth-factor supplement for sustaining cell growth and viability in SFM (Table 1).
Table 1: Independent studies showing LongR3 IGF-I superiority as a growth factor supplement
Table 1: Independent studies showing LongR3 IGF-I superiority as a growth factor supplement ()
Iron supplementation of SFM has also been demonstrated to be an essential requirement for mammalian cell growth by playing a role in DNA replication, cellular respiration, and metabolism. Lately, inorganic salts and chelators have been the products of choice to supply iron to cells in a serum-free media environment. However, to provide high-density cell cultures with sufficient iron, salts must be supplied at elevated concentrations that use low-affinity nonreceptor pathways. Iron salts at such levels can negatively affect cell growth when precipitation of insoluble ferric hydroxide forms in the medium, thus altering its composition and the bioavailability of iron to the cells. Unbound ferric ions can also be reduced by agents present in the media to form Fe2+, which promotes free-radical formation through the Fenton–Harber–Weiss reactions and potentially causes oxidative damage to the cells (18).
Transferrin is the natural and most efficient mechanism by which iron is delivered into cells (19). It was historically supplied as human (hTf) or bovine transferrin (bTf), but rTf is now available commercially. Expressed from baker’s yeast (Saccharomyces cerevisiae) as a recombinant analog of human transferrin, rTf has shown equivalence to hTf and industry relevant inorganic iron supplements and superiority to bTf for stimulating cell growth and productivity in mammalian cells, as in the “Superior Supplementation” box (12,20). Iron-loaded transferrin binds specifically to its receptor, where it is then transported into a cell through receptor-mediated endocytosis for efficient targeting to the mitochondria (7). Transferrin provides multiple benefits to a cell, acting as an extracellular antioxidant and binding iron so tightly that very little unbound iron is free to participate in free-radical formation.
Albumin is another supplement with great potential, given its very broad biological functions (2,24). The benefits of serum-purified albumin in cell culture are well documented (21,22,23). Because of its animal origins, the full potential of albumin as a media supplement has not been exploited. Recombinant forms of human serum albumin (HSA) already exist for a variety of biopharma manufacturing applications. An example recently launched by Novozymes Biopharma, CellPrime rAlbumin AF G (rAlbumin), was developed as a cell culture supplement specifically for the industrial market (24).
More recombinant bioactive factors will become available at a reasonable cost over time, so the potential for industrial cell biologists to reliably control cellular performance in culture could be better realized in the future. It may be possible that manipulation of multiple survival pathways in concert with cell cycle regulation for increasing protein synthesis or biomass further may be considered by the commercial sector as a viable process development pathway to reducing costs of biomanufacturing.
Recombinant Supplements in Combination
Because nutrient requirements for individual cell lines and even different clones of the same cell type vary considerably, it can be difficult and time consuming to design a single CD PFM for each production strain. Earlier studies at Novozymes Biopharma investigated the effect of the LongR3 IGF-I and rTf supplements on CHO performance, both alone and in combination. Productivity and growth were measured in the 96-well format described in the “Experimental Report” box, and a positive synergistic effect was observed when the two factors were used in combination. This synergistic response was also examined for other growth factors and iron supplements, as discussed in the “Synergy” box.
The potential for mammalian cell production of biotherapeutics is growing. Already levels of protein approaching 5–10 g/L in the production medium have been reported. This was unheard of just 10 years ago and has been achieved using a combination of growth-factor supplements and/or optimized feed strategies.
Biopharmaceutical customers are demanding a greater selection of animal-free supplements, and as this expands in the industry further improvements in expression levels may be achieved by combining technologies including animal-free growth factors, improved feeding strategies, and even genetic engineering. Moreover, in the future it may be possible to design a cost-effective supplement based on a range of animal-free components (chemical and biological in nature) that will offer the dramatic positive effects of FBS on mammalian cell growth without its associated negative attributes of high cost, variability of performance, and potential for contamination.
A Bright Future
The biopharmaceutical industry is constantly striving for optimal cell growth and productivity in upstream manufacturing processes while maintaining product/process consistency and regulatory compliance. Historically, the number of animal-free defined protein supplements available for use as industrial cell culture supplements has been limited. The rTf animal-free recombinant protein displays all the characteristics of its serum-derived counterparts without their associated regulatory concerns. Furthermore, it outperforms other inorganic sources of iron. LongR3 IGF-I growth factor is a proven cell culture supplement manufactured to the highest quality standards with performance that is equivalent (if not superior) to related growth-factor supplements. Data presented here also demonstrate the improved benefits that can be achieved through combined use of two biologically active protein supplements. Now, with the release of a recombinant albumin availability of defined, recombinant, animal-free, bioactive protein supplements specifically designed for industry is being addressed and allows media development scientists a robust alternative to protein-free media that deliver optimal performance to upstream manufacturing processes.
SUPERIOR SUPPLEMENTATION
BY SALLY GROSVENOR
To demonstrate the performance of recombinant transferrin (rTf) with respect to growth and productivity effects in a commonly used industrial cell line (CHO cells), studies were conducted to compare rTf with serum-derived hTf, bTf, and three inorganic iron salts. CHO clone 12 (expressing a human monoclonal antibody, MAb) was cultured in basal medium with 200 nM MTX and 2 mM Glutamax supplements and insulin. Before assay set-up, the cells were grown to 70–90% confluence and detached using trypsin. They were then washed and resuspended in serum-free, growth-factor–free medium. Cell viability was determined by the trypan blue exclusion method.
Each well of a 96-well microplate received 1,000 cells in fresh SFM. Plates were incubated at 37 °C in 5% CO2 and harvested at either day six or ten. On each harvest day, 100 µL of conditioned medium was removed to a separate plate for IgG analysis. The metabolic dye MTS was added to the remaining media for analysis of viable cell numbers, and absorbance was measured from each of six replicate wells to determine growth. The conditioned medium removed in the previous step was pooled for each test condition to create sufficient volume for three replicate analyses of IgG levels by ELISA (Figure 1). The results indicate that rTf and hTf were equipotent in stimulating CHO cell growth. However, cells treated with bTf showed little increase in cell growth by comparison with SFM. Moreover, CHO cells treated with rTf displayed growth and productivity profiles equal to or better than those treated with iron salts.
Figure 1: ()
SYNERGY: A BIOLOGICAL RESPONSE
BY SALLY GROSVENOR
To investigate the observed synergistic response of CHO cells treated with LongR3 IGF-I and rTf, the effect of replacing rTf with inorganic forms of iron in combination with the growth factor was tested. Results showed that combining the growth factor with rTf demonstrated the greatest response in increased cell growth and productivity compared with all other treatment combinations (Figure 1). Combining the growth factor with native hTf showed a similar response, whereas results of combining it with the bTf combination were poor, with little increase over growth factor treatment alone. Those results further support the hypothesis that a biological association causes the synergistic response — affinity for the TfR is known to be species specific (25). A lack of significant effects when inorganic forms of iron were combined with the growth factor is also consistent with the idea of a biological interaction involving the IGF-IR and TfR in the synergistic response (and that this is ligand dependent).
Figure 1: ()
To determine whether the enhanced performance was specifically a result of the LongR3 IGF-I combinations of rTf with related growth factors, insulin and IGF-I were similarly tested (Figure 2). Results confirmed that all members of the IGF family tested with rTf were able to elicit the synergistic response, which does suggest a role played by the IGF Type 1 receptor.
Figure 2: ()
REFERENCES
1.) Even, MS, CB Sandusky, and ND. Barnard. 2006. Serum-Free Hybridoma Culture: Ethical, Scientific, and Safety Considerations. Trends Biotechnol. 24:105-108.
2.) Merten, OW. 2002. Development of Serum-Free Media for Cell Growth and Production of Viruses/Viral Vaccines- Safety Issues of Animal Products Used in Serum-Free Media. Dev. Biol. 111:233-257.
3.) Schlaeger, EJ. 1996. The Protein Hydrolysate, Primatine RL, Is a Cost-Effective Multiple Growth Promoter of Mammalian Cell Culture in Serum-Containing and Serum-Free Media and Displays Anti-Apoptosis Properties. J.Immunol. Meth. 194:191-199.
4.) Hewlett, G. 1991. Strategies for Optimising Serum-Free Media. Cytotechnol. 5:3-14.
5.) October 2003. EMEA/410/01, Rev 2. Note for Guidance on Minimising Risk of Transmitting Animal Spongiform Encephalopathy Agents via Human and Veterinary and Medicinal Products. Off. J. European Union (2004/C 24/03).
6.) van der Valk, J. 2004. The Humane Collection of Fetal Bovine Serum and Possibilities for Serum-Free Cell and Tissue Culture. Toxicol. In Vitro 18:1-12.
7.) Zhang, AS. 2008. Use of Nramp2-Transfected Chinese Hamster Ovary Cells and Reticulocytes from mk/mk Mice to Study Iron Transport Mechanisms. Exper. Hematol. 36:1227-1235.
8.) Lok, CN, and TT. Loh. 1998. Regulation of Transferrin Function and Expression: Review and Update. Biol. Signals Recept. 7:157-178.
9.) Frasca, F. 2007. Role of c-abl in Directing Metabolic Versus Mitogenic Effects in Insulin Receptor Signalling. J. Biol Sci. 282:26077-26088.
10.) Xian, CJ. 1996. Production of a Human Epidermal Growth Factor Fusion Protein and Its Degradation in Rat Gastrointestinal Flushings. J. Mol. Endo. 16:89-97.
11.) Sunstrom, NAS. 2000. Insulin-Like Growth Factor-I and Transferrin Mediate Growth and Survival of Chinese Hamster Ovary Cells. Biotechnol. Prog. 16:698-702.
12.) Keenan, J. 2006. Evaluation of Recombinant Human Transferrin (DeltaFerrin™) As an Iron Chelator in Serum-Free Media for Mammalian Cell Culture. Cytotechnol. 51:29-37.
13.) Limke, T. 2008. CellPrime™ Recombinant Human Transferrin As an Animal-Free Alternative to Animal-Derived Transferrin or Iron Supplementation in Cell Culture Applications. BioProcess Asia-Pacific 1:48-49.
14.) Bertheussen, K. 1993. Growth of Cells in a New Defined Protein-Free Medium. Cytotechnol. 11:219-213.
15.) Castle, P, and JS. Robertson. 1999. Animal Sera, Animal Sera Derivatives and Substitutes Used in the Manufacture of Pharmaceuticals: Viral Safety and Regulatory Aspects. Dev. Biol. Stand. 99:191-196.
16.) Yandell, C. 2004. Vendor Voice: An Analogue of IGF-I — A Potent Substitute for Insulin in the Serum-Free Manufacture of Biologics by CHO Cells. BioProcess Int. 2:56-64.
17.) Buckler, A. 2007.The Effect of Genetically Modified Insulin Growth Factor on Cell Proliferation and Adenovirus Productivity in Serum-Free MediumCell Technology for Cell Products, Amsterdam, Springer Nederlands:619-622.
18.) Van Campenhout, A. 2003. Transferrin Modifications and Lipid Peroxidation: Implications in Diabetes Mellitus. Free Radic. Res. 37:1069-1077.
19.) Mackenzie, EL, K Iwasaki, and Y. Tsuji. 2008. Intracellular Iron Transport and Storage: From Molecular Mechanisms to Health Implications. Antiox. Redox Signaling 10:997-1026.
20.) Grosvenor, S. 2007. Enhance CHO Cell Performance with a Combination of CellPrime™ Recombinant Transferrin and LONG® R3 IGF-I. BioProcessing J. 6:45-51.
21.) Erkan, E, P Devarajan, and GJ. Schwartz. 2005. Apoptotic Response to Albumin Overload: Proximal vs. Distal/Collecting Tubule Cells. Am. J. Nephrol. 25:121-131.
22.) Ishima, Y. 2008. S-Nitrosylated Human Serum Albumin-Mediated Cytoprotective Activity Is Enhanced By Fatty Acid Binding. J. Biol. Chem. 283:34966-34975.
23.) Schiller, M. 2008. Hypothesis: Human Serum-Borne Albumin Bound Lipids Promote Cellular Survival After Apoptosis Induction By a Variety of Stimuli. Apoptosis 13:319-328.
24.) Keenan, J. 1997. Recombinant Human Albumin in Cell Culture: Evaluation of Growth Promoting Potential for NRK and SCC-9 Cells In Vitro. Cytotechnol. 24:243-252.
25.) Young, SP, and C. Garner. 1990. Delivery of Iron to Human Cells By Bovine Transferrin. Biochem. J. 265:587-591.