Using Innovation to Drive Competitive Advantage
September 1, 2009
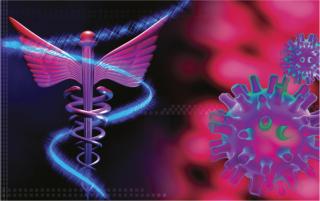
Figure 1: ()
STOCKXPERT (WWW.STOCKXPERT.COM)
After spending decades as the “sleepy” segment of the biopharmaceutical industry, vaccines are now seen as one of its highest growth segments. Major pharmaceutical companies — Novartis AG (www.novartis.com) and Pfizer, Inc. (www.pfizer.com), for example — are aggressively entering this area. Those already in it are expanding capacity and increasing business development activity. Indeed, access to the vaccines business was a major driver of Pfizer’s acquisition of Wyeth Pharmaceuticals (www.wyeth.com) (1).
Several factors are driving this change. Newly introduced vaccines are generating blockbuster revenues. Wyeth’s Prevnar accounts for nearly $3 billion of the company’s annual revenues, and Sanofi Pasteur’s Menactra (www.sanofipasteur.com) is approaching $1 billion. Concerns over influenza and bioterrorism and the potential for stable markets — both governmental and private — have made vaccines a more predictable business. An increasing recognition of the true public health value of vaccines, coupled with economic growth in emerging geographic markets, holds forth the promise of improved pricing and sales.
One consequence of all this increased interest has been a surge in funding directed toward novel vaccines and improved technologies. Over the past several years dozens of new biotechnology companies have been founded on the promise of new and better vaccines driven by innovative technologies. The major global vaccine manufacturers have also significantly increased their commitment to innovation.
In a previous article we reviewed the strategic and competitive challenges vaccine companies must meet in seeking to commercialize new vaccines (2). Here we profile some of the most promising novel technologies and suggest a means by which companies can use such innovations to drive sustainable competitive advantage.
Vaccine Innovation
The methods by which vaccines are developed and manufactured have changed surprisingly little since the creation in England of the smallpox vaccine by Edward Jenner in 1796. Only within the past two or three decades have novel technologies such as conjugation, recombinant technologies, and virus-like particle (VLP) methods found success. But the industry is likely to change dramatically over the next five to 10 years as many promising innovative technologies begin to reach the market. To know and appreciate where vaccine technologies are going, it is helpful to understand where they’ve been. So before reviewing some of the best of the new, we present a brief overview of the present in Table 1.
Table 1: Generations of vaccines
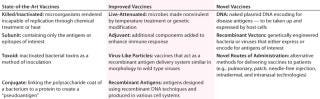
Table 1: Ge nerations of vaccines ()
The State of the Art
Current state-of-the-art vaccines include four major classes of product: killed or inactivated, subunit, toxoid, and conjugate vaccines.
Killed Vaccines: Early vaccines were developed by killing or inactivating the microorganism against which they were meant to immunize a patient. Killed or inactivated vaccines are rendered incapable of replication through treatment with chemicals such as formaldehyde or exposure to heat. This method has been used to fight bubonic plague, cholera, influenza, and hepatitis A.
However, the initial growth of such microorganisms can be time intensive. In the case of influenza, for example, a virus usually grown in eggs, the manufacturing process may take as long as 24 weeks. Additional reactogenicity is often associated with killed vaccines because all molecules are still present in dead/inactivated microorganisms — including their RNA and/or DNA.
Subunit vaccines were developed in an effort to eliminate such reactogenicity. These vaccines contain only the antigens or epitopes of a pathogen. Recently, such antigens or epitopes can be produced in multiple systems using recombinant technology, as with the hepatitis B vaccine produced in yeast. Subunit vaccines can contain multiple antigens or epitopes and are less likely to produce adverse reactions because they contain no additional molecules from the microbes. However, the cellular immune response to such vaccines is not as potent as for whole killed viruses.
Toxoid vaccines are composed of inactivated bacterial toxins. These were developed for bacterial infections that cause illness by releasing such toxins. The toxins can be inactivated with formalin and administered to patients as a vaccine. Toxoid vaccines are thus limited to those bacteria that cause illness this way, including diphtheria and tetanus.
Conjugate vaccines are developed by linking the poorly immunogenic polysaccharide coating of certain bacteria to a specific protein, which enables a patient’s body to recognize it as if it were a protein antigen. This approach is limited to bacteria with polysaccharide coats — such as pneumococcus, which is addressed by Wyeth’s Prevnar vaccine. Originally heptavalent, that product uses a diphtheria protein as a carrier.
Improved Vaccines
We call the next generation of vaccines “improved vaccines.” Largely the result of attempts to improve efficacy without sacrificing safety, they include live-attenuated vaccines, vaccines with adjuvants, virus-like particles (VLPs), and recombinant antigens.
Live-Attenuated Viruses: Live-attenuated viruses are nonvirulent and induce both humoral and cellular immunity, producing an immune response very similar to that of their wild-type counterparts. Viruses may be attenuated using temperature or through genetic engineering, such as NS1 manipulation of the influenza virus. Yellow fever, influenza, rubella, measles, mumps, and oral polio vaccine are examples of marketed live-attenuated vaccines.
Live-attenuated vaccines can be administered through multiple routes including intranasal, oral, or injection. Some evidence suggests that administration through the natural route of infection may improve the efficacy of these vaccines (3). Products with these routes of administration may therefore confer a significant advantage. However, because live-attenuated viruses are potentially capable of replication, such vaccines could mutate into virulent, disease-causing strains, which is why an inactivated vaccine is viewed as necessary for complete eradication of polio.
Adjuvants are additional components added to vaccines for boosting a recipient’s immune response. Although several successful vaccines have inoculated humans against harmful diseases, the trade-off between safety and efficacy has spurred the use of adjuvants.
Aluminum salts have traditionally been used in this role, but a more sophisticated attempt focuses on toll-like receptor (TLR) agonists and immunomodulators. The new generation of adjuvants under development largely targets the upregulation of cellular immunity by mimicking evolutionarily conserved molecular moieties of foreign pathogens, such as flagellum and other TLR ligands.
Adjuvants have recently attracted high amounts of investment because of their potential to improve efficacy in high–unmet-need patient groups such as influenza vaccination of the elderly. The ability to target specific subpopulations also positions such products for premium pricing. Several big pharma players have bet on adjuvants, including GlaxoSmithKline (www.gsk.com) through its acquisition of Corixa Corporation in 2005.
Despite their potential advantages, adjuvants also raise significant safety concerns. The correct pairing of an adjuvant to an antigen may be unique. As a result, one adjuvant may not be both safe and efficacious for more than one product. Consequently, it is likely that high levels of investment in clinical trials would be necessary for an adjuvanted vaccine — particularly for those innovative approaches being combined with novel vaccines.
Virus-Like Particles: Recently, VLPs have been developed and approved for use as vaccines. The technology is an antigen delivery system designed to induce both humoral and cellular immunity. VLPs resemble wild-type viruses in both form and elicited immune response but lack the genetic material necessary for replication. This provides a flexible system that can be manufactured in multiple cell systems and incorporate different antigens of choice. Such a platform might apply to a variety of novel vaccines, potentially offering improved efficacy and safety profiles.
Over the past decade, VLPs have been approved in two disease states: human papillomavirus (HPV) and hepatitis B. The recent commercial success of Merck’s Gardasil product (www.merck.com) has attracted investment and research into this area. VLPs can also be used to present foreign epitopes to a patient’s immune system, broadening their scope to potentially include immunotherapy for chronic diseases. The technology has been shown to induce autoantibodies and disease-associated self molecules involved in conditions such as hypertension and Alzheimer’s disease.
Recombinant Antigens: Recombinant DNA technology has enabled production of recombinant antigens that can be designed to specification and produced at large scale. The hepatitis B vaccine uses recombinant antigens, and the first such influenza vaccine, FluBlok brand manufactured by Protein Sciences Corporation (www.proteinsciences.com), is currently in phase 3 clinical trials.
Tomorrow’s Vaccines
Still other novel approaches to vaccines are in early stages of development. Technologies such as DNA vaccines, recombinant vectors, and alternative routes of administration address manufacturing and scalability needs — and in the case of alternate routes of administration, attempt to expand the acceptance of a vaccine product while still improving upon its efficacy.
DNA Vaccines: DNA vaccines use a microbe’s genetic material in plasmid form injected directly into a patient. The host cells are made capable of expressing the necessary antigens for immune response. Naked DNA is both cost efficient and scalable. Because DNA can be manufactured rapidly, this approach could be particularly useful as a response to a pandemic outbreak.
Adequate uptake of naked DNA into the appropriate cells is necessary to trigger an immune response capable of ensuring seroprotection. However, developing effective methods for delivering DNA have proven difficult. DNA-coated gold particles delivered by a pressurized helium gun is one such technique used by Powdermed (owned by Pfizer). But trials have shown that uptake of adequate amounts of DNA into the cells of interest has been a hurdle in larger-animal models. Using recombinant vectors to deliver DNA to host cells is another method being researched.
Recombinant Vectors: Vaccines using the genetic material of one microorganism combined with that of another to elicit an immune response are called recombinant-vector vaccines. Vectors can be attenuated viruses (e.g., the canary poxvirus, polio virus, or adenovirus) or bacteria (e.g., Salmonella and the BCG strain of Mycobacterium bovis). This method aims to exploit the efficiency certain viruses have for entering cells by using them as carriers of genetic information for other diseases. Alternatively, bacterial vectors are inserted with genetic material, causing them to display the antigens of other microbes on their surfaces. These methods are highly cost efficient and potentially scalable because multiple systems can be used to transfect or generate the vectors. Human immunodeficiency virus is one disease being targeted with this method.
Novel Routes of Administration: Vaccine innovation has traditionally focused on vaccine characteristics or antigen presentation. Alternative innovative approaches have focused on other areas of the vaccine value chain, including broadening the routes of administration. These methods aim to improve efficacy or expand patient acceptance. Some approaches may be dose-sparing, may induce a broader immune response, or may break the cold chain.
With patch formulations, lyophilized or dried antigens are coated on microneedles and applied topically for multiple hours. Active patch technologies involve heat, electroporation, or chemical activation to deliver an antigen from patch into host. Many are being used to deliver DNA vaccines. Although such vaccines themselves are inexpensive and quickly manufactured, the devices may prove costly and not as easily scalable. Companies such as Ioma/Intercell (www.iomai.com) and Althea Technologies (www.althea.com) have patch technologies entering the clinic.
Intradermal (ID) vaccines deliver a product directly to the dendritic Langerhans cells, direct activation of which focuses an immune response to those cells capable of initiating seroprotection, thereby improving immunogenicity. Thus far, ID approaches have faced difficulty ensuring accurate delivery of vaccines to those targeted cells.
Pulmonary vaccinations are helpful for diseases that use the respiratory system as a point of entry. Vaccination delivered to the lung mucosa can vary in technique, allowing for targeting of multiple different sites of the respiratory tract (such as deep lung or the upper or central airways), but they generally rely on aerosol generation technology.
Needle-free injection systems include powder-based, liquid needle-free pressurized injections, implants, and particle guns. These devices potentially offer safety and commercial advantages, but they may be costly to develop and are still in early stages of development. Both Inovio Biomedical Corporation (www.genetronics.com) and Pfizer (PowderMed) are developing gold-particle needle-free systems for delivery of DNA vaccines.
Other technologies in development include those focusing on breaking the cold chain, such as lyophilization, oral formulations, and nanoemulsions. With such a large number of technologies either improving existing vaccines or developing novel methods for inoculation, picking a “winner” among them takes more than good fortune.
Use Innovation to Drive Competitive Advantage
With the explosion in vaccine technologies, it is easy to be seduced by innovation for its own sake. But adoption and application of new technologies must be driven by their ability to generate revenues and contribute to the growth of a business by meeting important unmet needs. As vaccine manufacturers seek to derive competitive advantage by commercializing novel technologies, a structured process for doing so will be critical (Figure 1).
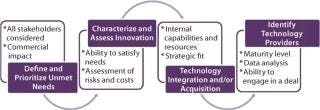
Figure 1: ()
Define and Prioritize Unmet Needs: For completely novel vaccines (e.g., for diseases with no current vaccines), the unmet need is usually clear: efficacy in preventing the disease. When considering next generations of existing vaccines, however, the unmet needs may not be as obvious — and they can vary by market or region. For example, there is significant need to improve efficacy for the elderly population. In considering vaccines for the developing world, the unmet need may focus on breaking the cold chain — that is, eliminating totally or in large part the need for refrigeration. In other cases, there may be opportunities to improve safety — as in development of acellular pertussis vaccines — or improve administration for ease or safety.
The challenge here is threefold: First, an unmet need must be viewed from the viewpoint of multiple stakeholders including patients, payers, regulators, and health authorities. Second, fulfillment of their needs should lead to significant commercial advantage through a combination of increased unit sales, premium pricing, improving penetration rates, or opening new markets. And finally, given that most vaccines will present numerous opportunities for improvement, some level of prioritization should be carried out that reflects (at a minimum) the commercial potential of the innovation.
Characterize and Assess Innovation Opportunities in Terms of Their Ability to Address Priority Needs: Once priority unmet needs have been identified, the next step is to identify, characterize, and select innovation types or classes that can contribute to meeting those needs. Accordingly, all relevant innovation classes must be identified based on knowledge of the industry, literature searches, and the like. To make this process efficient and logical, it can be helpful to array technology classes along the vaccine value chain (Figure 2).
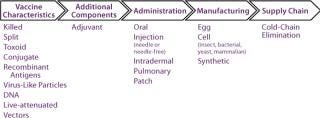
Figure 2: ()
Once a comprehensive inventory of technologies has been compiled, technologies should be arrayed and assessed against their ability to meet priority needs. For example, both traditional and next-generation adjuvants can enhance efficacy — but so can VLPs, viral vectors, and even alternative routes of administration. A selection/prioritization process will identify what factors would make a product stand out above the rest. Criteria such as technical feasibility, regulatory risk (and existence of a regulatory pathway), costs of acquisition and clinical development, and safety should be considered.
Consider Means for Acquiring/Integrating Desired Technologies: Companies have essentially two ways available to apply novel technologies: They can acquire them or develop them internally. In making such decisions, companies need to consider a number of factors: their internal capabilities and resources, the relative maturity of technologies internally and externally, the costs of “buying vs. building,” and the availability of willing external opportunities. A company’s evaluation of opportunities will be driven by its overall approach to using innovation. It can either buy “finished products,” or it can acquire technologies that it then uses to create products on its own. Both types of examples have been mentioned here.
Identify Specific Technology Providers and Initiate Business Development Activities: Once a company has decided upon the technologies it wishes to integrate, the final step is to define specific companies or other sources of those technologies (unless they will be developed internally). Depending on the technologies under consideration and their stage of maturity (preclinical, phase 1, and so on), they should be assessed against criteria that will include quality of available data, regulatory risk, and ease of acquisition. When promising sources have been identified, then a company can transition to a more traditional business development mode and explore acquisitions, partnerships, or licensing deals to find the one that best meets the needs of both parties involved.
Vaccines Are Hot
These are exciting times in the vaccine industry, with an increased awareness of the need for and benefits of these products. Novel vaccines are able to command prices related to their true value and become blockbusters. Organizations such as the Global Alliance for Vaccines and Immunization (GAVI, www.gavialliance.org) and the Bill and Melinda Gates Foundation (www.gatesfoundation.org) are providing unprecedented levels of support for both the development and administration of vaccines. Innovation is occurring faster than ever before — but innovation for its own sake will not have long-term impact. It must be adopted and applied in service of unmet needs and commercial benefit. Applying a structured process as outlined above will enable vaccine companies — both current and potential new entrants to the industry — to capitalize on innovative technologies for the benefit of themselves and the populations they wish to serve.
REFERENCES
1.) Singer, N. 26 January 2009.Wyeth, Pfizer Sees a Drug PipelineNew York Times.
2.) Allary, C, M Riché, and M. Edwards. 2008. Global Vaccine Commercialization: Competitive Challenge. BioProcess Int. 6:S4-S11.
3.) Katz, JM, S Garg, and S. Sambhara Kawaoka, Y. 2006.Influenza Vaccines: Current and Future StrategiesInfluenza Virology Current Topics, Caister Academic Press, Norfolk.
You May Also Like