Noninvasive Optical Sensor Technology in Shake Flasks
In process development, appropriate scaling is important to achieve acceptable product quality without compromising titer (1). Scale-down approaches involve matching the oxygen transfer coefficient (kLa) value, impeller tip speed, power per unit volume, or mixing time to those of a bioreactor (2). Bench-top bioreactors are typically used in bioprocess engineering as scale-down models of commercial units in fermentation and cell culture because of their similarity in geometry (H/D ratio) and mechanical properties (agitation type and sparging). By contrast, shaking culture systems such as Wave bioreactor (GE Healthcare), shake flasks, and tube spins are typically used for culture expansion.
Significant improvements to the composition, shape, size, and caps of shake flasks have facilitated sufficient oxygen transfer and mixing (3). Such modifications have allowed for use of shake flasks in production cultures for clone evaluation, media development, and process optimization. But discrepancies in cell culture performance with respect to growth, lactate, and productivity (which could be attributed to differences in pH and dissolved oxygen, DO) are frequently observed between shake flask and bioreactor cultures (4,5). So further improvements are needed before shake flasks can be more reliably used scale-down models for process development.
PRODUCT FOCUS: PROTEINS, ANTIBODIES
PROCESS FOCUS: UPSTREAM, PRODUCTION
WHO SHOULD READ: PROCESS ENGINEERS, QA/QC
KEYWORDS: PAT, PROCESS OPTIMIZATION, SCALE-DOWN, KLA, fed-batch culture, pH, dissolved oxygen
LEVEL: INTERMEDIATE
Although pH in shake flask cultures can be measured offline, it is not convenient to monitor it routinely. Sensor technology has evolved from fiber-optic probes (which are fragile when mechanically stressed and require autoclaving) to noninvasive sensors that can be affixed to sterile vessels (6). Advances in noninvasive fluorescence sensor technology have enabled the ability to monitor pH and DO values at a small scale. Methods for determining pH and DO values using noninvasive sensors include frequency-modulated excitation, fluorescence lifetime, dual-lifetime reference, and ratiometric measurement (7,8).
Sensor patches have evolved and are now <1 mm in size. Costs of sensors and patches have dramatically decreased, and they are widely used on a range of vessel types (9). Several commercially available systems use noninvasive sensors complemented with online monitoring capability for different types of cultures. Models include the PreSens Shake Flask Reader (SFR) system (Precision Sensing GmbH) and SENSOLUX stand-alone version (Sartorius Stedim Biotech) for shake flasks and Micro-24 miniature bioreactor system (Pall) and ambr miniature bioreactors (TAP Biosystems). In addition, the same technology has been used at larger scale in the Wave bioreactor and the Single Use Bioreactor (SUB) (HyClone) system.
Here, we compare performance of cell cultures run in shake flasks and bioreactors and present the necessity for pH and DO monitoring and control. We selected the PreSens SFR shake flask online monitoring system to investigate culture pH and DO differences among shake flasks and bioreactors.
Table 1 compares capabilities and costs of the PreSens system with those of a typical shake flask system and a 2-L bioreactor. Although it is more costly, online monitoring functionality warrants the additional cost. We tested the stability and accuracy of the pH and DO sensors to determine instrument functionality and calculated kLa values for shake flasks under different operating conditions.
Table 1: Comparing a traditional shake flask system, a PreSens shake flask system, and a 2-L bioreactor system
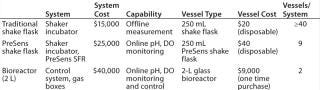
Table 1: Comparing a traditional shake flask system, a PreSens shake flask system, and a 2-L bioreactor system ()
We proposed operating conditions to match kLa values at large scale. We monitored the pH profile after bolus base addition (the traditional method of pH control in shake flasks) to determine the extent of the addition’s influence on culture pH. We examined the effect of a lack of agitation during shake-flask sampling on pH and DO profiles. Results elucidate the potential differences in shake flask cultures compared with bioreactor cultures.
Materials and Methods
The PreSens SFR system is a noninvasive online system for monitoring pH and DO for as many as nine shake flasks. The presterilized, precalibrated polycarbonate baffled shake flasks with vented caps have two immobilized sensor spots — one for pH and one for DO — affixed to their interior flask bottoms. The shake flasks are clamped onto the SFR, which houses an integrated optical module per shake flask for wireless data transmission to a PC for collection and visualization (Figure 1). The DO sensor uses a phase-modulation technique that correlates the luminescent decay time with a quantitative value of DO, calculated with the Stern-Volmer equation (8). The pH sensor uses an internal-referenced measurement with a patented combination of fluorescent dyes to detect changes in intensity and correlate it with a pH value. Given ranges for sensor detection are 0–100% air saturation for DO and 5.5–8.5 for pH.
Equation 1:
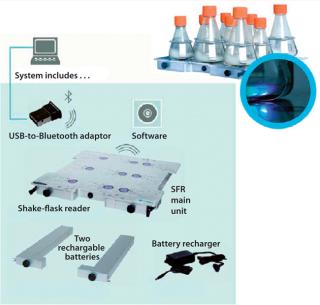
Figure 1: ()
Stability and Accuracy: We tested the stability of the pH sensor by monitoring the change in pH value of phosphate buffered saline (PBS) in a 250-mL shake flask over 12 days with a 15-second interval online sampling frequency. To determine pH sensor accuracy, we compared the online pH readings from the optical sensor with offline measurements from a calibrated Nova BioProfile 400 system (Nova Biomedical). We tested a 6–7.6 pH range by using 1N HCl and 1M NaOH to adjust pH values of the proprietary medium. We tested DO sensor accuracy
by comparing online values from the optical sensor to the air percentage setting of the shake flask incubator, termed “offline” in the figures. The percentage was changed through the mixed gas inlet by variation of the CO2 percentage in the incubator. A two-point calibration at 0% and 100% was achieved by sparging air into phosphate buffered saline (PBS) with and without sodium sulfite.
kLaStudies: We determined oxygen mass transfer coefficients (kLa) using a proprietary chemically-defined medium in 250-mL shake flasks. We used JMP software to design a full- factorial design of experiments (DoE) with varying working volumes (40, 80, and 120 mL) and shaker speeds (100, 150, and 200 rpm). Each condition was executed in duplicate, and the shake flasks were on a platform shaker with an orbital diameter of 19 mm.
We evaluated various kLa measurement methods (data not shown), and chose a method on the basis of consistency and data reproducibility. Briefly, a medium-filled shake flask was sparged with nitrogen gas (N2) until the medium was depleted of oxygen. This medium was transferred to an empty shake flask, which was then placed on the SFR and orbitally agitated in a 37°C incubator to monitor the DO increase until saturation was reached. We calculated kLa values using the linear portion of the online DO data according to the equation ln(C* – C)=kLa(t), in which kLa is the oxygen transfer coefficient, t is time, C is the oxygen concentration, and C* is the saturated oxygen concentration. We analyzed and graphed results in JMP.
Cell Culture, Equipment, and Assays: We used a suspension Chinese hamster ovary (CHO) cell line from Genentech, Inc. that expresses a therapeutic monoclonal antibody, (MAb A or MAb B) to show culture performance differences in shake flasks and bioreactors. We used a CHO cell line expressing a different monoclonal antibody (MAb C) for the bolus base addition and simulated sampling experiments. Those cell lines are derived from a dihydrofolate minus (DHFR-) host that uses DHFR/methotrexate selection (10). We thawed cells and maintained them in a proprietary selective chemically defined medium for two to three weeks. We transferred cultures to a proprietary, nonselective, chemically defined medium for a two-stage inoculum train stage before entering the production stage using the same medium. A day 3 feed consisted of 20% w/v of a proprietary chemically defined feed media.
We used a Forma Scientific incubator (Thermo Scientific) and an Innova platform shaker (New Brunswick Scientific) with an orbital diameter of 19 mm for shake-flask cultures. Culture conditions were 37°C with 5% CO2 and 85% humidity. Agitation occurred through orbitally shaking of the 250-mL shake flasks at 150 rpm. Applikon 2-L bioreactors controlled with a DeltaV BioNet system (Broadley-James) produced MAb A and MAb B. Sparging with air, oxygen, or a combination through an open pipe controlled DO, and CO2 and/or 1M sodium carbonate additions controlled pH. The initial temperature was 37°C, with a shift to 35°C on day 3; pH set-point was 7.0; and agitation of the pitch-blade impeller was 275 rpm. We used a Vicell AS (Beckman Coulter) system to obtain viable cell counts and the Nova BioProfile 400 unit for offline pH sampling.
Bolus Base Addition for pH Control: After bolus base addition, we monitored pH profiles to determine how long pH could maintain at the postbolus addition level in shake flasks. The culture used was a day 3 production culture and had a low pH of 6.6. We tested three conditions using 250-mL shake flasks with 100-mL working volume. The control flask was not pH-adjusted, one flask was adjusted to pH 7.0 and one flask to pH 7.3. We monitored pH profiles over 24 hours with a 15-second sampling interval. We used 1M sodium carbonate for the pH adjustments, and calculated the base addition amount with Equation 1 (11). Vadd is the sodium carbonate volume to add; Vtotal is the total culture volume; PCO2 is the partial pressure of CO2 in the culture; kH is the Henry’s Law constant for CO2 (value at 37°C used, 36 (L atm)/mol). [HCO3–]add is the carbonate concentration in the liquid base; pHf is the final pH; pHi is the initial pH; and pKa is the first dissociation constant of sodium carbonate base (value used was 6.3).
Simulated Sampling Procedure: Using four 250-mL shake flasks, we evaluated the effect of a simulated shake flask sampling procedure on pH and DO profiles. The control flask contained media only. Cultures of various viable cell densities (1×106/mL, 5×106/mL, and 10×106/mL) seeded the other three flasks. Agitation was stopped after 10 minutes of shaking at 150 rpm in the incubator, and we left the incubator door open to simulate manual sampling. Thirty minutes later, the incubator door was closed and agitation returned to 150 rpm. Monitoring of both pH and DO took place during this time period.
Results and Discussions
Shake flasks are used for clone selection and process optimization as a high-throughput, less expensive alternative to bioreactors. For MAb A, a pH of 7.0 maintained in the controlled environment of bioreactors compared with the uncontrolled shake flask environment, in which pH drifted to 6.75 corresponded with higher lactate levels. Cell density and titer reached in the bioreactor compared with those in shake flasks (Figure 2). In such cases, culture performance in shake flasks may not correctly predict performance in bioreactors. Such profile differences, however, are not present for MAb B, which showed no difference in pH for the shake flask culture compared with bioreactor culture. If pH and DO control were possible in shake flasks, they may be a better model for bioreactors in process development.
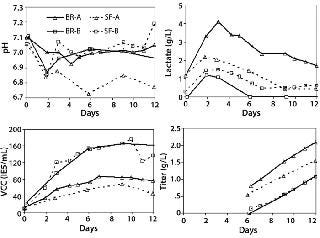
Figure 2: ()
Sensor Stability and Accuracy: We evaluated pH sensor stability because photobleaching occurs with the luminescent dyes in the sensors over time. The pH sensor drift over 12 days was 0.18 pH units — calculated to be 0.00275 units per 1,000 measuring points, which was close to the 0.003 units per 1,000 measuring points stated by PreSens. For pH sensor accuracy in the physiological pH range of 6 to 7.6 tested, the offset set to zero, the slope was 1.0 with an R2=0.93 for 55 data points (Figure 3A). The percent difference between offline and online pH readings was calculated using %difference=[(pHoffline – pHonline)×100] ÷ (pHoffline).
All values fell within 4% (Figure 3B), and >96% of the values fell within 2%. For the online readings, 86% were within 0.1 pH unit of the offline value, and 96% were within 0.15 pH unit.
The DO sensor drift stated by PreSens as ∼0.006% per 1,000 measuring points was not explicitly tested due to the negligible expected drift. D
O sensor accuracy showed online versus “offline” results with a slope of 1.01 and R2=0.99 for 20 data points (Figure 2C). We calculated the percent difference between online and “offline” DO values similarly to the pH percent difference, and it fell within 4% (Figure 2D). This value is considered a minimal difference in DO because changes in the 10–100% range have a negligible influence on culture performance (12).
Applications in Shake Flasks: Online monitoring capabilities of the PreSens SFR system can lead to improved process knowledge in shake flasks. Three applications discussed here are
gathering accurate kLa data based on online DO values to adjust shake flask rotation speed and working volumes to better match shake flask to bioreactor kLa values
determining the effectiveness of bolus base additions as a method for pH control in shake flasks
monitoring the impact of manual shake flask sampling on a culture to suggest an optimal sampling method.
kLameasurement: Results analyzed in JMP and shown in a contour plot represent the kLa profile. Figure 3 shows the values ranged between 3 and 16 1/h for the parameters tested: 80–120 mL shake flask working volume and 100–200 rpm shaking speeds. Under typical operating parameters for the 250-mL shake flasks of 80-mL working volume and 150 rpm, the predicted kLa values fall between 8 and 12 1/h (center section in Figure 4). We used a different method, which relied on color change based on sulfite oxidation (13), to confirm kLa values under the same operating conditions. That method provided a kLa value of 15 1/h (unpublished data), which was in the same range as JMP predicted.
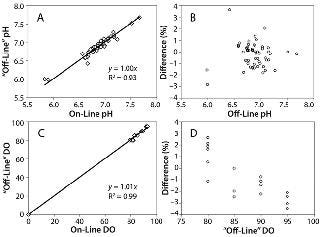
Figure 3: ()
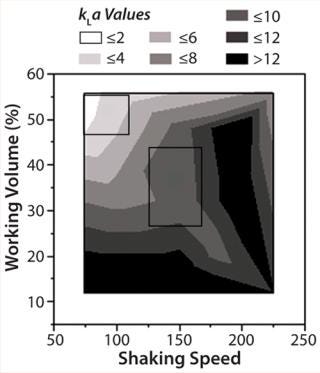
Figure 4: ()
Ries et al. (2010) published shake flask kLa values of up to 40 1/h using water in glass flasks (versus media in plastic flasks in our study). The higher value could be attributed to a difference in the method of measuring kLa (in their method, air is directly sparged into the headspace of the shake flask containing liquid that was out-gassed with nitrogen) (14). In our study, media out-gassed with N2 was transferred to a new shake flask.
In general, typical bioreactor operating conditions for cell culture provide kLa values of 2–4 1/h. To mimic values achieved in bioreactors, the shake flask operating range should fall in upper left section of Figure 4, which means lowering shaker speed and increasing shake flask working volume from typical operating conditions. In particular for 250-mL shake flasks, working volume should be 120–150 mL and shaker speed 75–100 rpm. We ran experiments with media only using the recommended conditions to mimic bioreactor kLa values. Our results confirmed the predicted kLa values (data not shown).
Effect of Manual pH Adjustment: Typically for shake flask operations, pH is controlled only by the medium’s buffering capacity and CO2% in the incubator. For cultures that trend toward low pH values, however, daily manual bolus base additions can control pH. Historical shake flask data from Genentech, Inc. show that pH in shake flasks can reach as low as 6.0, whereas pH in bioreactors is typically controlled at a value between 6.8 and 7.2. This low pH in shake flasks has been shown to affect culture growth and productivity when compared with bioreactors (4).Monitoring the online pH profile after bolus base addition helps determine the effectiveness of this pH control method.
Here, we inoculated the control flask with culture at a pH of 6.6 and adjusted cultures in the other two flasks to pH 7.0 and 7.3 by adding sodium carbonate. In the control culture, the pH dropped to <6.4 within an hour (Figure 5). The pH of the culture adjusted to pH 7.0 dropped to <6.8 in 20 min and to 6.6 in two hours. The pH of the culture adjusted to pH 7.3 dropped <6.8 in an hour and to 6.6 in 15 h. Therefore, the effectiveness of manual pH adjustment to maintain pH between 6.8 and 7.2 lasted for less than an hour for this culture. Bolus base addition is not an ideal method for pH control in shake flasks, especially in lactogenic cell lines that lead to low pH, because multiple additions would be required per day to maintain pH >6.8. Better methods of pH control in shake flasks need to be explored. Potential solutions include increasing media buffering capacity by using dual buffer systems or implementing slow-release technology for base addition (15).
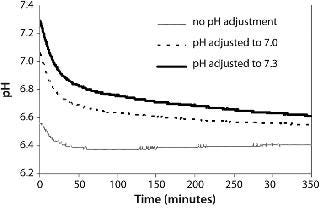
Figure 5: ()
Effect of Simulated Manual Sampling on pH and DO: The effect of shake-flask sampling on the culture had not been previously monitored, and it was assumed that minimal changes occurred in its physical properties. We monitored pH and DO changes during simulated manual sampling using the SFR (Figure 6) system.
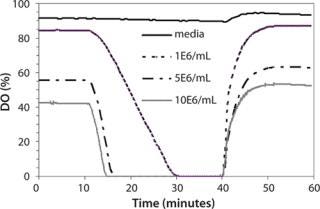
Figure 6: ()
The medium-only case and cultures of three cell concentrations (1×106/mL, 5×106/mL, and 10×106/mL) had starting DO values ranging from ∼90% to 40% because of their different oxygen demand. After 10 min with the incubator door open to simulate manual sampling, there was no change in DO in the medium-only case. DO decreased to 0% in 18 min for the 1×106/mL case and in four to five minutes for the 5×106/mL and 10×106/mL cases. Over the 30-min monitoring period, there was no change of pH for the medium-only case or the 1×106/mL case (data not shown). By contrast, pH dropped 0.3 pH units (observed repeatedly) for the higher cell densities (5×106/mL and 10×106/mL) in that time period, which could be attributed to one of many possibilities. For example, there could be decreased pCO2 stripping from the shake-flask culture lacking agitation, the cells could be producing lactate in response to the anaerobic condition, or the reading could be localized due to cells settling from lack of agitation. When the incubator door was closed and shaking resumed, it took less than two minutes for DO to reach above 30% and six to eight minutes for DO to reach original values for all culture-containing conditions. At the same time, pH returned to original values within two minutes.
Based on the pH and DO excursions we observed, the recommendation for shake-flask sampling is to control the time without agitation to less than five minutes per flask, especially for high-density cultures that may be sensitive to pH and DO decrease. An alternative solution would be to use shake flasks with attached culture sample lines that can be sampled while the flasks remain shaking inside an incubator. That could help decouple the effects of no agitation and the open incubator door during shake-flask sampling.
Comparable Performance
Culture performance in shake flasks — typically used in culture expansion and clone evaluation — can vary when compared with bioreactors. Therefore, monitoring parameters such as pH and DO is critical to determine the factors of variability. We found that the PreSens SFR system provides accurate online monitoring for pH and dissolved oxygen in shake flask cultures with all values falling within 4% of offline values. The kLa value for typical cell culture shake flask operating conditions is 8–12 1/h, which should be sufficient to meet oxygen requirements for cultures. Values similar to those of bioreactors (2–4 1/h) can be achieved with 250-mL shake flasks at shaking speeds of 75–100 rpm and working volumes of 120–150 mL. Further confirmation that this oxygen transfer rate is acceptable and that comparable culture performance and product quality to bioreactors achievable should be tested in shake flasks with culture.
Results presented here indicate that bolus base addition to adjust pH to 7.0–7.3 for pH control can only maintain our culture pH above 6.8 for less than an hour. So, frequent additions would be required, which diminishes the benefit of using shake flasks. The amount of time for shake flask culture to be without agitation during routine sampling should be kept to <5 min to prevent oxygen depletion, especially in high-density cultures. Capabilities of the PreSens SFR system provide those insights to shake flask cultures and in monitoring pH and DO, provided better understanding of their limitations in clone selection and process optimization. Monitoring can also lead to methods of optimizing their usage as a small-scale model to bioreactors.
About the Author
Author Details
Wen-Lin Tsai is engineer Iat La Jolla Biologics. Jennifer L. Autsen is an associate scientist IIof oceanside biologics at Gilead Sciences. Julie Ma is a student at the University of Colorado at Boulder in the department of chemistry and biochemistry. Terry Hudson is an associate director of process development engineering at Genentech. Corresponding author Jun Luo, PhD is a manager of Vacaville Manufacturing Science, Cell Culture at Genentech, 1000 New Horizons Way, Vacaville, CA95688; [email protected].
REFERENCES
1.) Li, F. 2010. Cell Culture Processes for Monoclonal Antibody Production. mAbs 2:466-477.
2.) Hu, F 2004. Scale-Up and Scale-Down on Cell Culture Bioreactors. Cell. Bioprocess Technol.:214.
3.) Humphrey, A. 1998. Shake Flask to Fermentor: What Have We Learned?. Biotechnol. Prog. 14:3-7.
4.) Yuk, IH.
5.) Kostov, Y. 2000. Unique Oxygen Analyzer Combining a Dual-Emission Probe and a Low-Cost Solid-State Ratiometric Fluorometer. Appl. Spectrosc. 54:864-868.
6.) Buchs, J. 2001. Introduction to Advantages and Problems of Shaken Cultures. Biochem. Eng. J. 7:91-98.
7.) Tolosa, L. 2002. Noninvasive Measurement of Dissolved Oxygen in Shake Flasks. Biotechnol. Bioeng. 80:594-597.
8.) Kostov, Y. 2001. Low-Cost Microreactor for High-Throughput Bioprocessing. Biotechnol. Bioeng. 72:346-352.
9.) Hanson, MA. 2007. Comparisons of Optical pH and Dissolved Oxygen Sensors with Traditional Electrochemical Probes During Mammalian Cell Culture. Biotechnol. Bioeng. 97:833-841.
10.) Urlaub, G, and LA. Chasin. 1980. Isolation of Chinese Hamster Cell Mutants Deficient in Dihydrofolate Reductase Activity. Proc. Natl. Acad. Sci. 77:4216-4220.
11.) Chen, A. 2008. Twenty-Four Well Plate Miniature Bioreactor System as a Scale-Down Model for Cell Culture Process Development. Biotechnol. Bioeng. 102:148-160.
12.) Oh, SK. 1992. Further Studies of the Culture of Mouse Hybridomas in an Agitated Bioreactor with and without Continuous Sparging. J. Biotechnol. 22:245-270.
13.) Herman, R. 2001. Optical Method for the Determination of the Oxygen-Transfer Capacity of Small Bioreactors Based on Sulfite Oxidation. Biotechnol. Bioeng. 74:355-363.
14.) Ries, C. 2010. A Shaken Disposable Bioreactor System for Controlled Insect Cell Cultivations at Milliliter Scale. Eng. Life Sci. 10:75-79.
15.) Jeude, M. 2006. Fed-Batch Mode in Shake Flasks By Slow-Release Technique. Biotechnol. Bioeng. 95:433-445.
You May Also Like