Construction and Start-Up Costs for Biomanufacturing Plants
All bioproducts heading to commercialization go through a rigorous process that includes research and development, proof of concept, and validation studies. Furthermore, the fabrication process of a biological product is very different from that of a classical (synthetic small-molecule) pharmaceutical. That’s not only because of specific characteristics and requirements of the molecular entities involved, but also environmental considerations and specific issues related to human health and safety. Such differences are reflected in the cost drivers of approved manufacturing plants.
Herein we identify those cost drivers by examining real-life examples of biological products manufactured in Canada. Specifically, we contrast cost drivers of biopharmaceuticals and industrial bioproducts, especially with regard to current good manufacturing practice (CGMP) regulations. We examine a CGMP plant and two CGMP-compliant plants. The data are compared with those and an industrial bioproduct (bioethanol) for which there are no GMP requirements. Our analysis was preceded by in-depth interviews with consultants specialized in the building and validation of CGMP bioplants. Because the study was done in Canada, all costs presented here are in Canadian dollars.
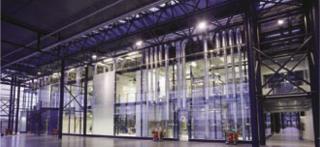
The authors explore the monetary differences between building GMP-compliant bioprocessing facilities (such as this award-winning Novo Nordisk plant in Hillerød, Denmark) and other types of biomanufacturing facilities. ()
Definitions
Concepts involved in this article are defined briefly as follows. The term GxP is a generalization of quality guidelines, predominantly those used in the pharmaceutical industry. The purpose of such guidelines is to ensure product quality, guiding pharmaceutical research, development, and manufacturing. The most central aspects of GxP are traceability (the ability to reconstruct the full development and manufacturing history of a drug) and accountability (the ability to resolve who has contributed what to that history, and when).
Good manufacturing practices (GMP) are standard guidelines set out by the US Food and Drug Administration (FDA) to ensure that drug development is carried out in safe and high-quality processes to prevent contamination and ensure repeatability. The term current good manufacturing practices (CGMPs) emphasizes that the expectations are dynamic and is used to ensure that products made will meet specific requirements at the time the work is done.
Hazard analysis and critical control points (HACCP) is an internationally recognized food safety system used to help ensure the manufacture of safe food products. It provides a more systematic approach to ensuring food safety than traditional inspection procedures, and it places more responsibility for ensuring food safety on food manufacturers than traditional inspection programs.
Context
Before discussing our cases studies, we first briefly review their technical, legal, regulatory, and socioeconomic contexts.
Technical Context: Settling on a single definition of the term bioproduct is increasingly difficult as technological advances generate an ever-greater diversity of products derived from biological sources. The case studies presented here illustrate this clearly through examining biopharmaceuticals, biologically derived medical devices, and an industrial bioproduct. Specific technical issues and hurdles vary according to the product and its associated manufacturing process, with some important similarities and differences.
Currently the term biopharmaceutical equates almost exclusively with recombinant therapeutic proteins and/or engineered antibodies. These are typically produced using a cell-based expression system of bacterial, fungal, or mammalian origin. The choice of cell system is based on characteristics of the product itself, such as its size, complexity, and requirements for posttranslational modifications (particularly glycosylation). Production systems for biopharmaceuticals include Escherichia coli as well as engineered animal cell lines (mainly Chinese hamster ovary, CHO, and baby hamster kidney, BHK, cells). Nine of the 31 therapeutic proteins approved since 2003 are produced in E. coli,. whereas 17 are produced by mammalian cell lines. Engineered Saccharomyces cerevisiae yeast is also used for production of a limited but important group of biopharmaceuticals, most notably recombinant hepatitis B surface antigen (HBsAg, GlaxoSmithKline) and insulin (NovoNordisk).
Major technical issues for biopharmaceuticals, especially those produced using mammalian cell culture, involve the need for high product yield and consistent and correct glycosylation of the protein. Over the past decade a clear focus has improved existing production systems to provide >10-fold increases (from 250 mg/L to 2 g/L or more) in product yield through cell line and process optimization. A number of alternative production systems also have been developed, and those capable of carrying out correct glycosylation include yeast, insect, and plant cells. Compared with mammalian cell lines, such cells typically grow to higher densities in shorter fermentation cycles using less expensive and more chemically defined media, and they offer a lower risk of transmitting mammalian pathogens.
Of course alternative production systems, whether cell culture or fermentation or transgenic organisms, are not limited to biopharmaceuticals. Industrial bioproducts can also be made using such systems as well as others that are not generally used for therapeutic products: e.g., bacterial species other than E. coli, a large number of different yeasts and filamentous fungi, and plants. Mammalian cell culture is not a process of choice for industrial bioproducts because it is technically complex, slow, and expensive and is not mandated by the nature of the products themselves. As with biopharmaceuticals, one major technical issue is that of product yield.
Bioethanol is an interesting example that includes two separate biological transformation steps. The first is the use of enzymes (e.g., amylases and cellulases) required to release fermentable sugars from a feedstock. Corn is most frequently used in North America, although great efforts are going into research of a wide range of other cellulosic feedstocks. The necessary enzymes are produced by a number of different organisms that have increasingly been genetically modified to improve enzymatic performance under specific process conditions. Increased performance equates to a more efficient conversion of a given feedstock to fermentable sugars — and therefore more efficient ethanol production. Efforts are also being made, as with biopharmaceutical production systems, to increase the titer of enzymes expressed.
The second transformation step in bioethanol manufacture is alcoholic fermentation whereby yeasts produce ethanol from the sugars. The focus here again is on improving the efficiency of conversion by using yeast strains that have been selected or genetically modified to have a higher tolerance to ethanol or to ferment a wider variety of sugars.
Such technical considerations significantly affect the overall costs for manufacturing bioproducts in th
at they dictate certain requirements of the production processes. However, they are only a few of the many factors to take into account in facility planning.
Legal Context: Different standards must be respected according to legal requirements, especially regarding architectural plans and environmental aspects. Generally the construction of a manufacturing plant is controlled by municipal standards that review (and hopefully accept) that project.
In biomanufacturing, some legal aspects must be considered that depend on the product itself. For example, in a bioethanol plant, a production permit is required by the Canada Revenue Agency because ethanol is an alcohol, and denatured alcohol must meet the specifications set out in the regulations for denature alcohol (1).
The environmental aspect is another point to consider in constructing a manufacturing plant. The Canadian Environmental Assessment Act constitutes a legal basis for the federal process of environmental evaluation (2). The Canadian government is using such evaluation as a tool for planning to determine, understand, evaluate, and attenuate (if possible) the effects of a project on the environment. Under the terms of the Act, federal ministries and related organizations must provide environmental evaluation of a project when a federal authority is providing funds or any other form of financial assistance for the project’s realization. According to article 20, it is important to ensure that a construction project is unlikely to cause major, detrimental environmental effects.
Regulatory Context: For biopharmaceutical development and manufacturing, technical know-how is essential as well as knowledge of the latest technology and global regulatory requirements, including those of the US Food and Drug Administration (FDA), Canada’s Therapeutic Products Directorate (TPD), and the European Medicines Evaluation Agency (EMEA). Because the United States represents the biggest market, and its regulatory requirements can be considered as a standard, we cover only FDA requirements here.
In GMP facilities, regulation of the production and laboratory testing environment itself is extremely important. Sampling products statistically ensures only that the samples themselves (and the areas adjacent to where they were taken) are suitable for use. End-point testing relies on sampling. A crucial instrument of GMPs is documentation for all aspects of a process and its activities and operations. If the documents are not correct and in order, showing how a product was made and tested and allowing for traceability and recall from the market in the event of future problems, then the product is considered contaminated or adulterated.
In GMP manufacturing plants, the air entering a cleanroom from outside is filtered to exclude dust, and the air inside is constantly recirculated through high-efficiency particulate air (HEPA) and ultralow-penetration air (ULPA) filters to remove internally generated contaminants. Employees enter and leave through airlocks (which sometimes include an air shower stage), and wear protective clothing such as hats, face masks, boots, and cover-alls. Equipment inside a cleanroom is designed to generate minimal air contamination.
Low-level cleanrooms often are not sterile (free of uncontrolled microbes), and more attention is given to airborne particles than to surfaces. Particle levels are usually tested using a particle counter. Some cleanrooms are kept at a higher air pressure so that in the event of a leak, air will rush outside. It is similar to the lower pressure used in biological “hot zones” to keep dangerous microbes inside. Cleanroom HVAC systems often control humidity to low levels such that extra precautions are necessary to prevent electrostatic discharges.
Social and Economic Context: The health-care biotechnology industry has mushroomed over the past decade (with revenues increasing from $8 billion Canadian in 1992 to over $39 billion in 2003), and it shows no sign of slowing (3). Some 165 biopharmaceuticals have now gained medical approval, and an estimated 300–700 protein-based therapeutics are at various stages of clinical development. By 2010, analysts predict up to a further 50% increase in total approved product numbers, with a total market value of $52 billion (compared with the total value of $34 billion in 2004) (4). Treatments are emerging from clinical trials ready for marketing and scaling up for commercial production, which will lead to a growing need for new facilities.
Biotechnology is not uniquely dedicated to health care however, and the past decade has also seen a surge in industrial biotechnology. Innovations have led to cleaner processes that produce less waste and use less energy and water in such industrial sectors as chemicals, pulp and paper, textiles, food, energy, and metals and minerals. In 2005, the Biotechnology Industry Organization (BIO) reported that industrial biotechnology (including products made from biobased feedstocks or through fermentation or enzymatic conversion) accounted for 7% of sales and $100 billion in value within the chemical sector. Growth was expected to continue, with those values reaching 10% of sales and $160 billion in value by 2010.
Methodology
To better understand the challenges in building a biotech factory, we met with engineering consultants at three different firms who specialize in design and construction of such assets. Each meeting consisted of a one-hour semistructured interview. Each time, we supplied a hypothetical manufacturing process to standardize our collected information. We also interviewed personnel from three biotechnology companies with production facilities. The first three interviews enabled us to gather a better understanding of the cost drivers in attaining CGMP compliance.
Finally, we built a case study for a factory making bioethanol, a product without a CGMP obligation. For this, we used several Internet sources for documentation (e.g., 5)as well as interviews with government officials. Our aim was to replicate as closely as possible the costs (direct and indirect) of a bioethanol plant and thus understand the cost drivers for a non-CGMP industrial unit for comparison with CGMP-compliant facilities.
We submitted a single model to the consultants to standardize their response. The drug substance we wished to “produce” is a highly purified recombinant human enzyme for use in enzyme-replacement therapy. The final product was further defined as a sterile, nonpyrogenic, lyophilized powder for reconstitution with sterile water for injection from USP.
Each estimate should thus take into account the creation of a 10,000-ft2 facility for dedicated CGMP process manufacturing and quality control. The model should also include the following critical preprocess activities: receipt and release of raw materials; receipt, sampling, and storage in a quarantine-controlled area; testing the samples against specifications and issuing certificates of analysis; then finally moving raw materials into a released, controlled area where they are stored until their use in manufacturing. Stringent environmental controls are needed. Cell culture inoculation and production occur in class 100,000 clean rooms (with air changed through HEPA filters 45 times per hour), purification and filtration steps occur in class 10,000 clean rooms, and fill–finish steps occur in a class 100 clean room. A detailed manufacturing process for Chinese hamster ovary cells (CHO cells) stably expressing recombinant enzyme at 2 g/L was also posited.
Consulting Firms
We discussed general aspects with three consulting firms. Estimates are based on square footage corresponding to a Class D evaluation. To obtain an estimated cost, it is necessary to multiply the plant surface by a factor that depends on the purpose of an area. The area can be a clean
room, a laboratory, an office, or a warehouse. Table 1 lists the multiplying factors per area for the different consulting firms.
Firm A’s intervention is generally in engineering, procurement, and construction management. It estimates that the space in a 10,000-ft2 plan would be divided as follows: clean rooms 30%, laboratories 15%, airlocks 5%, offices 25%, mechanical room 10–15%, and conditioning 10%. Several additional areas should be considered when designing a plant: a ventilation system, a plumbing system, an ultrapure water system, the different airlocks (for weighing, for the instruments), a premix room, a room to put or remove overalls, cloakrooms, corridors, an office inside the cleanrooms for the person responsible for them, and pressurization toward the outside (100 particles of 0.5 µm per cubic foot inside the clean room, 10,000 particles/ft3, and even 100,000 particles/ft3 outside the clean rooms). Architectural plans, consultants for the frame, and mechanical and electricity consultants correspond to 10% of the final cost. Firm A estimated that about one year would be needed to complete the proposed 10,000-ft2 biopharmaceutical plant.
Firm B is an engineering group specializing in the design of pharmaceutical, food and beverage, and industrial plants. It offers the following services: process engineering, validation, mechanical and electrical engineering, process development support, process equipment integration engineering in buildings, automation, process-oriented project management, and energy studies.
According to Firm B, 25% of a biopharmaceutical plant surface is dedicated to manufacturing and 75% to support (offices, warehouse, QC laboratory, and mechanical and electrical rooms). These consultants said that 40% of “soft cost” should be added onto the manufacturing direct costs if all GMP-related processes are considered, e.g., validations, controls, and training. The cost of a process is generally an investment equivalent to the substructure. Non-GMP plants have between 40% and 60% of manufacturing area and a minimum of support (about 20% of soft cost). Time from construction to start-up would be 18–36 months for a biopharmaceutical plant and 6–12 months for a non-GMP plant.
Firm C is an engineering and construction firm focused on the life sciences sector. It also provides services and solutions in R&D, scale-up, manufacturing, packaging, and distribution. With the help of a biological process engineer from this firm, we determined the costs associated with construction, installation, and validation of a turn-key facility based on our model production design.
A plant such as our model was estimated to require a 12–15,000-ft2facility with two floors, the first floor dedicated to production, mechanical support, and utilities (electricity and plumbing) and the second housing office space and quality control (QC) laboratories. It was suggested that the warehouse be a separate building altogether because a CGMP facility requires controlled entry and exit of all items and personnel.
In addition to the cost of the CGMP shell, an estimated $300/ft2 was calculated for process equipment based on our model. Another $120/ft2 (40%) of the cost needs to be included for equipment installation. Initial validation of the facility, performed between installation and the first production runs, is estimated to be 5% of the total cost of a plant and its process equipment.
According to the different consulting firms, a number of CGMP-specific elements were highlighted that contribute to the total cost of a biomanufacturing plant. They are listed in the “GMP Infrastructure and Operations” box.
Case Studies
Company A has created and developed innovative biotherapeutics for regenerative medicine (tissue repair) and therapeutic delivery. It is preparing its own production facility, with analytical and research laboratories (20,000 ft2). The company’s quality management system is registered ISO 9001:2000, which indicates compliance with international regulatory requirements for design, development, and manufacturing.
GMP INFRASTRUCTURE AND OPERATIONS
Infrastructure
Different operations must be segregated in different rooms, which are connected by doors or through airlocks.
Air handling is by separate HVAC units for different cleanroom classes.
Floors need to be in “bath-tub” style (with drains), and their finish needs to be resistant to the strongest cleaning agents.
All equipment surfaces contacting the product must be made of special 316-grade stainless steel, for which there are a limited number of approved suppliers.
Operations
To prevent cross-contamination, only one batch of product can be made in any one room at any one time.
A minimum of two operators must be in each room all the time to ensure that correct procedures are followed.
Any item entering or exiting the facility must be recorded and monitored.
In addition, it was mentioned that filling and finishing for the model type of product is extremely difficult to perform from an operational standpoint because they require maintenance of the most stringent cleanliness conditions in the entire facility (a class 100 cleanroom). For this reason, filling and finishing are often contracted out to specialty companies.
The new plant will be a 10,000-ft2unit (two floors of 5,000 ft2 each) with clean rooms (aseptic filling, clean vapor), five offices, and some extra space. About 20% of the surface is allocated to GMP production, whereas 80% is allocated to non-GMP rooms. The cost is estimated to be $350/ft2 for the non-GMP space and $600/ft2 for GMP clean rooms. Here are the different costs related to the building, equipment, engineering, and validation, and finally the start of the plant: building $4 million, equipment and installation $1 million, and engineering and validation $1 million, totaling $6 million. Equipment and installation includes a water system, autoclave, depyrogenation oven, aseptic filling machine (which costs $300,000 alone), and other instruments. The filling machine has a low capacity of 10,000 units/day (1,000 units/hour).
The company expects about a one-year delay for final construction and validations. Other expenses will be incurred before manufacturing, particularly employee salaries, maintenance costs, and validation of the processes every three months. Many QC employees are required in this manufacturing plant because of the complexity of its processes. The estimate of these costs (with a workforce of 11 employees) is as follows:
Production director $120,000
Production technicians (four) $40,000–60,000 each
QC supervisor $80,000–100,000
Four QC technicians at $40,000–45,000 each
Quality assurance (QA) director $80,000–100,000
Maintenance costs (cleaning, repairs, external validation) $400,000–500,000
Total $760,000–925,000.
All required analytical assays will be performed in-house: identification, purity, sterility, pyrogenicity, stability, mechanical tests, batch release, and raw materials testing. Results must meet established specifications of each batch, and 25% of the full manufacturing costs relate to QC testing.
The main cost drivers for this case study are costs related to filling and finishing. Because flasks must be kept sterile, and their manipulation must be aseptic, tremendous costs are incurred in following CGMP packaging procedures. That is in addition to the many costs related to extra personnel needed for ensuring compliance.
Company B is a supplier of native marine collagen. Its innovative technology produces ultrapure native collagens in significant supplies from various raw material sources. The company has a 3,000-ft2 production facility that, although it is not CGMP-approved, was designed with GMP compliance in mind. About 80% of the surface is allocated to production, with 20% for non-CGMP rooms (e.g., storage). The facility already has ISO 9001:2000 approval. Direct costs are estimated as follows: building $67/ft2 ($200,000), equipment and installation $327/ft2 ($980,000), and coordination and expertise $83/ft2 ($250,000), totaling $447/ft2 ($1,400,000).
The building was a basic multipurpose structure constructed by a local economic development agency. To comply with GMP requirements, the company built a second shell inside that building, then added its own equipment, which includes air and water purification systems, the collagen producing equipment, and a refrigeration system. Operating costs with a workforce of seven employees were identified as follows:
Production director $65,000–75,000
Assistant director $35,000–45,000
Four production technicians at $30,000–40,000 each
QC technician $40,000–50,000
Maintenance costs (cleaning, repairs, external validation) $75,000–100,000
Recurring costs (heating, electricity, taxes) $24,000–24,000
Total $359,000–454,000.
The QC technician has 100% of his time allocated to quality control, whereas the other personnel have 5–10% of their time invested in quality control. Provincial and municipal norms were not prohibitive. Because the product made at the facility (collagen) is well known, the permits required were limited to demonstration of competent waste disposal. The facility uses no solvents or chemicals that carry a risk of explosion, which further reduced related norms and regulations.
For this company, the greatest cost drivers related to GMP certification were found in the cost of equipment. To reduce validation and inspection costs, all equipment purchased was CGMP-certified and installed by its manufacturer following CGMP guidance. So the white rooms (cleanrooms), extraction tanks, ventilation system, and water purification were bought and installed for CGMP compliance. Costs were appropriately higher than they would have been otherwise. For example, the ventilation system covering a 1,500-ft2 clean room cost $120,000.
Company C focuses on the development, production, and commercialization of protein-based biopharmaceuticals using a proprietary manufacturing system developed from its expertise in the genetic engineering of plants. This company has a $14,000-ft2 factory that includes a 10,000-ft2 biosafety level 2 greenhouse and a primary recovery suite used for treatment of biomass, along with a purification suite (a class 100,000 clean room). The rest of the space is allocated to production and some offices. The plant is CGMP-compliant but does not currently make CGMP products. Direct costs are as follows: building $1,800,000, equipment and installation $300,000, and coordination and expertise $900,000, totaling $214/ft2 ($3,000,000).
The square-footage cost of this factory is relatively low due to its large greenhouses. Maintenance and cleanup are completed by internal staff. No uncertified personnel are allowed in the greenhouses. Operating costs with a workforce of six full-time employees and eight part-time employees (20% production, 80% R&D) were identified as follows:
Two supervisors at $80,000–90,000 each
Four technicians at $40,000–50,000 each
Total allocated budget $64,000–80,000
QA team $150,000–200,000
Supplies for technicians (50% of total salaries) $267,000–330,000
Recurring costs (heating, electricity, taxes, security) $390,000–390,000
Total $657,000–720,000.
No prohibitive environmental municipal and provincial laws apply to this facility. All waste is filtered, no dangerous substances are used, and there are two sedimentation basins on site. The main cost drivers related to CGMP compliance come from allocated personnel. There is already a team on site that supervises and enforces CGMP guidance, and over 40% of its time is dedicated to documentation and insurance compliance. If the company obtained CGMP certification, it would need an extra technician. As for machinery and building costs, even if this company decided to shift to a non-CGMP strategy, its costs would be the same for compliance with HACCP regulations. The only difference would be in the purification process and the ventilation system.
Company D (Simulated): Some existing factories and those being built in Canada use either corn (Ontario and Quebec) or wheat and barley (the prairie provinces) to produce bioethanol. The main focus of our interview centered on existing plants, especially the Varennes, Quebec, factory being built by Commercial Alcohols, Inc. (www.comalc.com) that began operation in February 2007. That factory uses corn, has an annual capacity of 125,000,000 L, and required an investment of $100,000,000. Also discussed was the Suncor Energy Inc. (www.suncor.com) factory, which opened 31 August 2006 in Sarnia, Ontario. It uses corn, has an annual capacity of 200,000,000 L, required an investment of $120,000,000, and presently employs 38 people.
Besides financing costs (often described as 20% equity and 80% debt structure) and taxes, there were three main cost drivers for a bioethanol factory. Raw materials (cereals) account for 70% of operating costs. Energy accounts for 20% (in Canada, natural gas is the main source). And other products (e.g., chemicals) account for 4.5% of costs. Other costs included personnel (35 employees representing 3% of total costs) and upkeep and transport (2.5%). Hence, operating costs are driven by three factors that have been known to fluctuate.
As for capital, development, construction, and start-up costs for a new ethanol factory, these vary by period, building site, size and type of raw material (e.g., corn or wheat). And the following elements cannot be forgotten: design, engineering, construction (80%); and land, project development, legal and administrative costs, human resources, financial costs, and overhead (20%).
The costs of developing and commissioning a plant using cereals as raw materials can range from $0.75/L of production capacity for a factory with a yearly capacity of 200,000,000 L up to $1.50/L of production capacity for one with a yearly capacity of 60,000,000. As a result, we believe cost-drivers are the size of the industrial unit, raw materials, energy, and chemical products used. On average, a factory producing 120,000,000 L/year is estimated to cost $100,000,000 to build.
Discussion
Although each consultant approached our model facility differently and focused on various aspects of either infrastructure or process, they all agreed on the cost drivers. First and foremost are the CGMP regulations and guidelines, which mandate specifications for infrastructure, equipment, and process operation. Respect of these norms entails an enormous cost for the construction and operation of a compliant facility, but it is difficult to estimate what percentage of the total overall cost can be attributed to GMPs. The specific process is of course the secondary cost driver because it mandates specific raw materials, equipment, and operation costs.
That can be illustrated simply by comparing a biopharmaceutical produced using E. coli with one produced using CHO cells, as in our model process. Bacterial fermentation occurs over a few days, uses simple fermentation media, and requires few if any antibiotics because the fermentation time is short and bacterial cultures are more resistant to contamination than eukaryotic cells. By contrast, mammalian cells must be maintained over several weeks, are fed with expensive culture medium (probably supplemented with various vitamins, growth factors, and antibiotics), and are more susceptible to contamination and growth failure.
CHOOSING COMPLIANCE
During the course of our study, we found that some companies chose CGMP compliance for reasons that went beyond strict regulatory requirements. The main reason that they comply with such regulations (even if they were dealing with a product, such as a neutraceutical, which did not require it) is to gain a commercial advantage. Most have found that it adds an extra level of quality assurance to a product (as an ISO certification does).
Compliance also can be part of preemptive planning when faced with evolving government regulations, which tend to be increasingly severe. In addition, CGMPs prepare a plant for future production of pharmaceutical products, for which margins are often much higher than those in the nutraceuticals industry.
Clearly the decision to become CGMP compliant when it is not strictly mandated must be part of a larger strategic plan. Factors such as the size of a facility and the complexity of its production process will directly affect the cost of regulatory compliance, so this strategy may be most suitable for small facilities and/or relatively simple processes.
In the drug industry, adoption of more efficient technologies and processes to decrease costs poses a major challenge because it is limited by regulatory constraints that lead to a situation of playing perpetual technological “catch-up.” An old maxim, “The process is the product,” still holds true for most biotherapeutics: Any change to the production process requires a major amendment to be filed with the FDA and/or other regulatory agencies. Most companies therefore prefer to stick with their processes originally certified for commercial use, even if newer and more economically advantageous technological developments are available. Such advances are reserved for the next generation of new products in development.
By contrast, industrial bioprocesses are not subject to such stringent regulatory constraints and can therefore be modified more readily to incorporate new technologies. Their determining factors are more straightforward and strictly economic: The time and money necessary to implement an advance must be largely offset by increased process efficiency.
For each of the companies we interviewed, the main cost drivers for their new industrial units were related to compliance with GMP regulations. For Company B, the cost driver for its factory was purchasing and installing CGMP-compliant water and air purification. For Company A, it was the specialized packaging equipment and personnel. And for Company C, the maintaining of a specialized QA team as well as the dedication of up to 30% of the time of other factory personnel to quality control accounted for a major part of its annual costs. Hence, regulatory compliance — be it through certified equipment or a specialized team or other related element — becomes the main cost driver for companies building and maintaining CGMP facilities.
Cost drivers are completely different for an ethanol manufacturing plant. Production of bioethanol is weakly regulated and therefore does not face such constraints as a major cost factor. Rather, its cost drivers are similar to those of other industrial product manufacturing considerations and include infrastructure capital costs determined by the capacity of a facility and raw materials, energy, and chemical products used. As for other commodities, profit margins are rather small and added values are low, especially when compared with biologic drugs. Profit margin can be increased significantly with volume due to economies of scale, something that is less pronounced with biopharmaceuticals. For example, a scale-up process that would double the production capacity of a bioethanol facility would require only a fraction of the initial cost.
In summary, we examined specific factors that explain why CGMP compliance is a complex and expensive constraint on the biopharmaceutical industry. Although non-GMP biological products have similar cost structures to traditional manufacturing products, CGMP-compliant facilities often have distinctive cost-drivers. All things considered, costs will undoubtedly increase in the future due to increasingly higher regulatory requirements, and companies will need to carefully assess their overall process to properly understand all related costs.
REFERENCES
1.) Canada Revenue Agency EDN5: Denatured and Specially Denatured Alcohol Regulations.
2.) Canadian Environmental Assessment Act 1992.Canada Department of Justice.
3.) Biotechnology Industry Facts 2007., Biotechnology Industry Organization, Washington.
4.) Pavlou, A, and J. Reichert Recombinant Protein Therapeutics: Success Rates, Market Trends, and Values to 2010. Nat. Biotechnol. 22:1513-1519.
5.) (S&T)2 Consultants Inc., and NorrisPenny Meyers. 2004. Economic, Financial, Social Analysis and Public Policies for Biodiesel: Phase 1, Natural Resources Canada.
You May Also Like