A Decade of FiltrationA Decade of Filtration
June 1, 2012
Filtration is just as vital to bioprocessing as chromatography — and arguably even more so. Filters are not only used as downstream unit operations in themselves, but also in support of nearly every other step in bioprocessing. Gas or liquid filters ensure the quality of incoming air and feeds for cell culture operations, clean the circulating media in perfusion processes, aid in harvest clarification, and remove buffers from chromatographic eluate after chromatography columns. They are used in formulation work and fill–finish, as well as in environmental monitoring and process waters. And in the 21st century, filters have expanded their utility even more with the advent of chromatographic membranes.
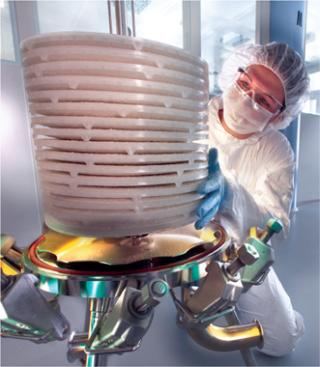
This photo of a technician setting up a filtration operation graced the cover of BPI’s November 2006 issue. ()
Disposability
Filter cartridges were among the first disposable items available for use in bioprocessing. In fact, filter manufacturers have led the charge toward single use — in many cases merging with other companies known as pstream players along the way (e.g., Sartorius and Stedim) to cover the whole manufacturing process with single-use options. From BPI’s first supplement focused on disposables (1,2,3,4) through the mainstreaming of single-use technology into regular issues (6,7,8), filtration has led the way from fixed stainless steel systems to polymer-based equipment. Experience with polymer-based filter materials even provided both vendors and users with a starting point for leachables/extractables testing of other polymer components as part of new types of qualification and process validation (4, 5, 8,9,10,11,12,13,14). Virus/Sterile Filtration
One area in which filtration is particularly indispensable is viral safety (15,16,17,18). Known and/or unknown viruses (even in minute numbers, each particle just tens of nanometers in size) can severely damage an entire process stream. Micro-and ultrafilters — e.g., Asahi-Kasei’s Planova brand, Sartorius Stedim Biotech’s Virosart brand, EMD Millipore’s Viresolve brand, and Ultipore filters from Pall Life Sciences — provide robust virus reduction, but no filter is perfect. Some (even just one) of the very smallest viruses may permeate due to even slight variations in pore size distribution. Nanotechnology could offer an option in the future.
Sterile filtration has other uses beyond virus clearance (19,20,21,22). Among them is removal of Mycoplasma bacteria, which lack cell walls and are thus unaffected by most antibiotics. Several species are pathogenic to humans (e.g., M. pneumoniae, which causes respiratory disorders, and M. genitalium, believed to be involved in pelvic inflammatory diseases). These smallest known microbes are ∼0.1 µm in diameter, so they can be removed only by membranes rated to that pore size (or smaller). Millipore Express, Pall Fluorodyne and Supor, and Nalgene PES filters are some of the available options.
Pall and Q-One Biotech partnered in 2003 to offer filtration as a possible means of dealing with prion risk (19). However, over the past decade, most biopharmaceutical companies have preferred instead to use media and other raw materials that are serum free, animal product free, protein free, and chemically defined wherever possible. Membrane Chromatography
Membrane adsorbers combine typical filtration action with chromatographic binding ability (23,24,25,26,27). Familiar brands include ChromaSorb from EMD Millipore, Mustang from Pall, and Sartobind from Sartorius Stedim. Available chemistries cover the range of liquid chromatography formats familiar to bioprocessing from ion exchange to reverse-phase to hydrophobic interaction — and even some affinities, although I haven’t yet seen a protein A version. Evaluating Filters
Clearly downstream process engineers have many options available for addressing the new manufacturing bottleneck that advancements in production technology have created for them (28,29,30,31,32,33). And BPI has sought to help them navigate the maze of types and brands and sizes and materials on the bioprocess market since the beginning. Different approachesapply to membranes (34,35,36) and depth filtration (36).
Integrity testing helps filtration experts determine whether a filter can operate within or outside validated specifications to provide a sterile effluent (37,38,39,40). Nondestructive testing may be performed on filters before and after use. The most commonly used types of tests performed on hydrophilic filters, for example, are bubble-point tests and diffusion tests. Here’s just one of many areas where automation is making inroads, as companies such as EMD Millipore and Sartorius Stedim Biotech offer specialized devices to facilitate the process (37, 40).
Q&A with BPI Authors: David W. Kahn and Maik W. Jornitz
David W. Kahn is senior director of the purification sciences department at Human Genome Sciences Inc., 14200 Shady Grove Road, Rockville, MD 20850; 1-301-610-5790, [email protected]. Maik W. Jornitz is senior vice president of marketing and product management in bioprocess solutions at Sartorius Stedim North America Inc., 5 Orville Drive, Bohemia, NY 11716; 1-631-870-8463; [email protected].
BPI: What were the early difficulties with single-use filtration?
DK: Many disposable filter options have been used from very early on in the history of the biotech industry, particularly depth filters and normal-flow filters. The early difficulties in implementing single-use devices were similar to hurdles the technologies face currently: specifically, concerns about the cost of filters when implemented at large scale, the possibility of toxic leachable components or molecules capable of direct damage to the product, and disposal of potentially large amounts of biowaste in the form of used filtration devices.
MWJ: Capsule filter devices were first available in smaller filter areas, but industry requirements required fully scalable filter capsules from 100 cm2 to nowadays 3 m2. Early filter capsules were heat sterilized by autoclaving, but their first connections to single-use bag assemblies required gamma-irradiatable polymeric housings to avoid elevated particulate and extractable release.
BPI: Are there any types of bioprocess filtration that don’t lend themselves as well to single-use?
DK: I believe that the greatest challenge in implementing single-use filters would be for membranes used in ultrafiltration and diafiltration steps. For this application, vendors currently provide multiuse products that are readily cleaned and reused, so the economic advantages of single-use filters decrease with our ability to get extended lifetimes (>20 uses) from existing cassettes. There is also an economy of scale; at this point, it’s not practical to imagine using disposable UF/DF cartridges at larger bioprocessing scales (> 5,000 L). Use of disposable UF/DF cassettes at least currently looks more appealing in manufacturing clinical products.
MWJ: The main restrictions are probably in large-volume filtration or high-contaminant filtration steps, which require larger surface areas that cannot be built into single-use devices. For example, large-scale media preparation still uses multiround filter cartridge systems (up to 12 round 30-in. filters). Those devices are installed into stainless steel housings. A few years ago, cell-harvest filter systems were also available only for installations within stainless steel housings, but new developments make a mu
ltitude of single-use systems available. These developments may be of help in designing large-scale sterilizing-grade filter systems in the future, which will be single-use and handle 20,000 L of cell culture media. Other (more rare) applications such as solvent filtration do not allow for single-use filter devices.
BPI: Membrane chromatography may be one of the most significant recent advances in “filtration” technology. Are there any biopharmaceuticals yet approved using this in their downstream processes, or do you know of any coming soon?
DK: I am not aware of a commercial process using membrane chromatography. That said, I would expect this would be right around the corner because ion-exchange membranes in particular appear to have overcome some of the early hurdles and look to have established themselves as one more useful tool in the process development toolbox.
MWJ: I would classify membrane chromatography rather as a separation or purification technology than filtration. It has been used in approved processes for over 10 years and finds a rising popularity due to speed and low buffer-volume needs. Multiple scientific papers show that membrane chromatography has economic advantage far above classical chromatography systems. Besides, membrane chromatography devices are single use.
BPI: What are currently the biggest/most common challenges in optimizing filtration steps for bioprocessing?
MWJ: The most common difficulty when performing optimization trials is the lack of test fluid volumes due to the fact that these fluids are extremely expensive and often needed for other trial work. This causes, in some instances, insufficient trial work that can lead to the wrong filter choice, long-term yield losses, and elevated running costs. Furthermore, process-scale systems must be sized up from 47-mm trial discs — truly only indicator trials that need to be backed up by verification trials using pleated devices. Thus, often a filter system is oversized, resulting in large hold-up volumes and elevated nonspecific adsorption and extractables. To prevent insufficient sizing and filtration process designs, it is advisable to use the support services of filter manufacturers, which have a multitude of test results in their databases that can help when filtration systems are newly designed.
DK: I still think a significant optimization challenge would be for virus filters to achieve an adequate balance between reducing cost (less membrane) and specifying enough of a safety factor to deal with the much more challenging feedstocks produced in small-scale spiking studies. Many people in the industry are working on creative solutions (e.g., cleaner virus preps and use of alternate models) that will help in this area.
BPI: And what do you think of as the most significant filtration-related technological advancement(s) over the past decade?
DK: If we’re looking back a full decade, I’d say the the tremendous advances made by multiple vendors in producing robust, scalable, high-capacity, high-flux virus filters that are capable of multiple-log reductions in parvovirus. We now take it for granted that this unit operation will perform exactly as intended when implemented.
MWJ: Looking at the sterilizing-grade filter introduction over the past decade, clearly the most significant development is in new polyethersulfone (PES) membrane designs. Of the past 21 sterilizing-grade filters launched, 20 contained asymmetric PES membranes. They show exceptional chemical, mechanical, and thermal resistance in combination with excellent flow rates and total throughputs. Nanofiber prefilters are another technological advancement that will enhance prefiltration steps to protect either sterilizing, virus, or tangential-flow filtration steps. Their fiber size is very thin, which allows for a denser filter matrix with higher flow rates and total throughputs than classical nonwoven filter materials. Optimizing Filtration
Choosing a filter — or filters — is only the beginning. Like every other unit operation in bioprocessing, filtration falls under the purview of quality by design (QbD). In conjunction with design space definition, process optimization has increased in importance over the past decade. And as vendors continue to offer new and improved membrane materials, users are finding ways to take advantage of those options for addressing the downstream bottleneck. Prefilters have an important role to play (41). And even advancements in sensor technology are helping (42).
Scaling up is certainly a top concern, and different types of filters involve different types of strategies and challenges (43,44,45). For example, Dave et al. observe that “lack of adsorptive capacity in a depth filter may lead to particle breakthrough during filtration or after exhaustion of adsorptive capacity when saturation of the depth filter is reached.. .. The consequence of adsorptive capacity is thus very important for filters downstream of the depth filter(s) because they can experience a sudden onset of particle load and foul suddenly…. Therefore, it is important to monitor the pressure on both the depth filters and filters downstream from them during small-scale experimentation because both modes of failure (pressure build-up and breakthrough) can occur independently” (43).
Q&A with an Expert
Leesa McBurnie is manger of laboratory services and validation at Meissner Filtration Products, Inc., 4181 Calle Tesoro, Camarillo, CA 93012; 1-805-388-9911, fax 1-805-388-5948; [email protected].
BPI: Our May 2006 issue had an article from Meissner on filtration validation. How has the approach changed in bioprocess organizations over the past 10 years? What kind of help do filter vendors provide?
LMB: With the first release of PDA’s Technical Report #26 in 1998 and its subsequent 2008 revision (which reiterated the validation approach and offered guidance on single-use systems), pharmaceutical and bioprocess organizations have a high degree of confidence in what the FDA expects for filter validation. Filter vendors typically have dedicated laboratories and staff to perform validation services and work closely with customers in assessing risk factors, documentation requirements, and testing protocols. Meissner staff members remain active on industry committees that continue to develop guidelines and best practices.
BPI: Your company also contributed to our very first supplement on single-use technology. We think of filtration as one of the first bioprocess areas where disposability was an option. What were the early challenges, and how were they met?
LMB: Small-scale capsule filters were available in a “single-use” format while the bioprocessing industry was still in its infancy. When first introduced, those devices were largely for convenience in eliminating external hardware and minimizing operator exposure to contaminants. Researchers in bioprocessing immediately adopted them for those reasons but had to take a relatively large number of components, autoclave them, assemble them aseptically, and then clean them (except for the single-use filter).
Customers loved the filters and soon adopted single-use components — such as biocontainers to replace stainless carboys — but were still assembling various components and managing sterility requirements. The significant breakthrough occurred when users could easily obtain complete fluid-patch assemblies that were sterile and validatable. Such systems are being increasingly scaled to larger sizes.
More recent challenges that we’ve addressed in developing our portfolio (e.g., biocontainer assemblies) involve
leachables and extractables (L&E) concerns. Meissner was the first company to eliminate slips agents such as erucamide and oleamide from its biocontainer films. Oleamide is a biologically active molecule. The ultimate ability to reduce leachables in single-use systems is predicated on excelling in polymer science and understanding materials of construction.
BPI: What do you think is the most significant filtration-related technological advancement over the past 10 years?
LMB: One of the most significant filtration-related advances has been the advent of large-scale, ready-to-use capsules with filters integrally sealed into plastic housings. Ten years ago, the largest devices contained ∼0.3 m2; today the largest devices contain 3.5 m2 or more (e.g., Meissner’s UltraCap HD capsule filters). We have creatively eliminated the time and expense associated with change-out of filters from traditional stainless steel housings and their associated cleaning and reassembly as well as cleaning validation concerns. That lessens downtime between filtration runs and improves productivity. New process methods allow these filters to be manufactured in continuous lengths (10–50 inches) to support small filtration campaigns through large-scale production runs.
Another significant technological change is the advancement of high-flow, long-life filter membranes. For example, Meissner first introduced its sterilizing-grade STyLUX PES membrane filter over 15 years ago. Its asymmetric pore structure allows for extremely fast flow rates and low pressure drops. The asymmetry makes for a final filter with its own built-in prefilter. Meissner delivered the second-generation sterilizing-grade EverLUX PES membrane filter in 2005. It provides even better flow rates with lower pressure drops and longer life for extremely particulated fluids that tend to blind off other filter media.
Giglia and Sciola add, “Scaling predictions of large-scale membrane filtration device performance from that of small-scale devices must account for a number of factors,including differences in flow geometries between small-and large-scale devices, extra-membrane flow resistances, and nonidealities in fluid-accessible membrane area. Variability in membrane and fluid properties can also add uncertainty in scaling estimations” (45).
Membrane fouling reduces the performance of ultrafilter membranes. It has been an inevitable problem in protein processing, but some newer processes minimize or eliminate the problem hrough proper selection of, e.g., modified (stabilized) regenerated cellulose (M-RC) ultrafilters (46).
An experimental approach provides better understanding of any filtration process. Although many such processes share certain features, experts know that peak performance can’t be counted on without characterizing the actual product being filtered. Performing optimization studies maximize product yield, minimize process time, and economize equipment sizing (47).
About the Author
Author Details
Cheryl Scott is cofounder and has been senior technical editor of BioProcess International since the first issue; 1-646-957-8879; [email protected].
REFERENCES
1.) Bardo, B. 2004. Production-Scale Disposable Filtration in Biomanufacturing. BioProcess Int. 2:S44-S46, S50.
2.) Meyeroltmanns, F. 2004. Crossflow Filters Enter the Disposables Arena. BioProcess Int. 2:S48-S50.
3.) Haughney, H, and J. Hutchinson. 2004. A Disposable Option for Bovine Serum Filtration and Packaging. BioProcess Int. 2:S52-S55.
4.) Cappia, J-M, and NBT. Holman. 2004. Integrating Single-Use Disposable Processes into Critical Aseptic Processing Operations. BioProcess Int. 2:S56-S58, S63.
5.) Mills, GJ, and B. Bardo. 2006. Biopharmaceutical Filtration Validation. BioProcess Int. 4:30-38.
6.) Lentine, KR. 2006. Preparation of Redundant, Disposable Filtration Systems. BioProcess Int. 4:44-47.
7.) Nicholson, P, and E. Storm. 2011. Single-Use Tangential Flow Filtration in Bioprocessing. BioProcess Int. 9:38-47.
8.) Pegel, A. 2011. Evaluating Disposable Depth Filtration Platforms for MAb Harvest Clarification. BioProcess Int. 9:52-56.
9.) Carter, J, and H. Lutz. 2003. Validation of Virus Filtration. BioProcess Int. 1:52-62.
10.) Extractables and Leachables Subcommittee of the Bio-Process Systems Alliance 2008. Recommendations for Extractables and Leachables Testing. BioProcess Int. 6:44-52.
11.) Ding, W, and J. Martin. 2008. Implementing Single-Use Technology in Biopharmaceutical Manufacturing. BioProcess Int. 6:34-42.
12.) Bestwick, D, and R. Colton. 2009. Extractables and Leachables from Single-Use Disposables. BioProcess Int. 7:S88-S94.
13.) Pora, H, and B. Rawlings. 2009. A User’s Checklist for Introducing Single-Use Components into Process Systems. BioProcess Int. 7:9-16.
14.) Mire-Sluis, A. 2011. Extractables and Leachables. BioProcess Int. 9:14-23.
15.) Brorson, K, G Sofer, and H. Aranha. 2005. Nomenclature Standardization for “Large Pore Size” Virus-Retentive Filters. BioProcess Int. 3:S21-S23.
16.) Cabatingan, M. 2005. Impact of Virus Stock Quality on Virus Filter Validation. BioProcess Int. 3:S39-S43.
17.) Wu, Y. 2008. Validation of Adventitious Virus Removal By Virus Filtration. BioProcess Int. 6:54-59.
18.) Valax, P. 2009. Robustness of Parvovirus-Retentive Membranes and Implications for Virus Clearance Validation Requirements. BioProcess Int. 7:S56-S62.
19.) Sellick, I. 2003. Prion Clearance with Membrane Filters. BioProcess Int. 1:54-56.
20.) Priebe, P, and H. Bromm. 2005. Filtration for Protecting Cell Cultures. BioProcess Int. 3:72-74.
21.) Schroeder, HG. 2006. Sterility Assurance with Filtration. BioProcess Int. 4:38-47.
22.) Folmsbee, M, and M. Moussourakis. 2012. Retention of Highly Penetrative A. laidlawii Mycoplasma Cells Using a New 0.1-μm–Rated Membrane Filter at Elevated Pressure and at an Elevated Challenge Concentration. BioProcess Int. 10:60-62.
23.) Gottschalk, U, S Fischer-Freuhholz, and O. Reif. 2004. Membrane Adsorbers. BioProcess Int. 2:56-65.
24.) Mora, J. 2006. Disposable Membrane Chromatography. BioProcess Int. 4:S38-S43.
25.) Lim, JAC. 2007. Economic Benefits of Single-Use Membrane Chromatography in Polishing. BioProcess Int. 5:48-56.
26.) Pattnaik, P, I Louis, and MS. Mahadevan. 2009. Use of Membrane Technology in Bioprocessing Therapeutic Proteins from Inclusion Bodies of E. coli. BioProcess Int. 7:54-62.
27.) Fraud, N. 2009. Hydrophobic-Interaction Membrane Chromatography for Large-Scale Purification of Biopharmaceuticals. BioProcess Int. 7:S30-S35.
28.) Kossik, J. 2004. Plasmid DNA Purification Possibilities Using the Disposable Rotary Drum Filter. BioProcess Int. 2:72-73.
29.) Schwartz, L. 2003. Diafiltration for Desalting or Buffer Exchange. BioProcess Int. 1:43-49.
30.) Arnold, TE. 2005. Fluid Purification Using Charge-Modified Depth Filtration Media. BioProcess Int. 3:44-49.
31.) Tepper, F, and L. Kaledin. 2006. A High-Performance Electropositive Filter. BioProcess Int. 4:64-68.
32.) Meyeroltmanns, F, and M. Grabosch. 2007. Downstream Ultrafiltration for Human Serum Albumin. BioProcess Int. 5:56-59. https://bioprocessintl.com/wp-content/uploads/bpi-content/070305ar08_77293a.pdf
33.) Eschbach, G, and S. Vermant. 2008. TFF Membranes for High MAb Concentration. BioProcess Int. 6:66-69.
34.) Priebe, PM, MW Jornitz, and TH. Meltzer. 2003. Making an Informed Membrane Filter Choice. BioProcess Int. 1:64-66.
35.) Peters, S. 2007. A Simple Method for Evaluating Filtration Membranes. BioProcess Int. 5:50-55.
36.) Blanchard, MM. 2007. Quantifying Sterilizing Membrane Retention Performance. BioProcess Int. 5:44-51.
37.) Carter, H. 2008. Integrity Testing Low-Area Filters Using Air–Water Diffusion and an Automatic Integrity Tester. BioProcess Int. 6:62-65.
38.) Madsen, RE, TH Meltzer, and MW. Jornitz. 2010. How Pore and Fibrous Interstice Structure Influence Filter Performance, Part 1: Fluid Effects and Operations. BioProcess Int. 8:58-64.
39.) Madsen, RE, TH Meltzer, and MW. Jornitz. 2010. How Pore and Fibrous Interstice Structure Influence Filter Performance, Part 2: Particulate Effects and Experimental Findings. BioProcess Int. 8:48-54.
40.) Meyer, J. 2012. Integrity Testing of Sterilizing-Grade Filters. BioProcess Int. 10:54-58.
41.) Ireland, T, G Bolton, and M. Noguchi. 2005. Optimizing Virus Filter Performance with Prefiltration. BioProcess Int. 3:S44-S47.
42.) Clark, KJR, and J. Furey. 2007. Application of Disposable Pressure Sensors to a Postcentrifugation Filtration Process. BioProcess Int. 5:S62-S65.
43.) Dave, P, J Dizon-Maspat, and T. Cano. 2009. Evaluation and Implementation of a Single-Stage Multimedia Harvest Depth Filter for a Large-Scale Antibody Process. BioProcess Int. 7:S8-S17.
44.) Dosmar, M, F Meyeroltmanns, and M. Gohs. 2005. Factors Influencing Ultrafiltration Scale-Up. BioProcess Int. 3:40-50.
45.) Giglia, S, and L. Sciola. 2011. Scaling Up Normal-Flow Microfiltration Processes. BioProcess Int. 9:58-62.
46.) Dosmar, M. 2005. Could Membrane Fouling Be a Thing of the Past? BioProcess Int. 3:62-66. https://bioprocessintl.com/wp-content/uploads/bpi-content/0302ar09_77179a.pdf
47.) Dosmar, M. 2006. An Experimental Approach to Optimization of Ultrafiltration. BioProcess Int. 4:44-54.
You May Also Like