The Reoccurrence of Mycoplasma Contamination: Prevention StrategiesThe Reoccurrence of Mycoplasma Contamination: Prevention Strategies
The contamination of microbiological media by mycoplasmas such as Acholeplasma laidlawii is not a recent phenomenon. It has been a major problem with animal-derived sera since the 1980s and has been a concern in the management of cell cultures for decades. The main culprit of serum contamination was the inadequate blood collection methodology and was eliminated with the introduction of hollow collection needles. In addition to the introduction of an improved collection method, serum was filtered with 0.1 µm-rated membrane filters to isolate potential mollicute contaminants, albeit with varying degrees of success (1,2,3,4).
Improved collection and filtration technologies mitigated the mycoplasmas contamination problem, until the threat of spongiform contaminants in animal-originated media initiated a switch to plant peptone-derived cell culture media. One contaminant was exchanged by another, which, once again, turned out to be mycoplasmas. This time, however, to varying degrees, the contaminating species were not restricted to Acholeplasma laidlawii but also included Mycoplasma orale, pneumoniae, fermentans, arginini, salivarum, hominis and hyorhinis. Occurrences of mollicute contaminations started to rise and resulted in a significant problem for the biotechnology industry. Depending on the information source, the contamination rate of biotech secondary cell cultures varies between 15–30% and 1% of primary cell cultures in the US (5,6). It became necessary to devise strategies to prevent the described contaminants from entering the process stream, as mycoplasmas are a serious human pathogen and can alter cell expressions and/or target proteins. This paper describes some of the countermeasures that can be used to abate this contamination risk.
First Line of Defense: Detection
Without doubt, the most important preventive activity against any contaminant is detection. Once detected, specific strategies can be established to eliminate the contaminants. These activities should be utilized by the raw material supplier and, if necessary, by the end-user. However, the direct assay detection of mycoplasmas in accordance with FDA’s Points to Consider Document (7) is a rather slow process. The method asks for both, agar and medium inoculation; no less than 0.2 mL of product sample is spread over at least two agar plates and is incubated for at least 14 days at 36 ± 1 °C in an anaerobic atmosphere. Another sample >10 mL is introduced into 50 mL of medium (commonly Hayflick) and incubated. Samples of the medium culture are taken at days 3, 7 and 14 and sampled on agar plates. These agar plates are incubated as described above; thus, the minimum time to determine a contamination is 28 days. The need for such a long wait for the release of cell culture media is costly … and a major logistical headache. What’s worse, in a pandemic outbreak scenario, any vaccine release needs to be rapid and simply cannot afford a 28-day release period. Additionally, the traditional approach to the detection of mycoplasmas can be difficult to execute and requires experienced personnel.
Parallel to the direct agar/broth method, an indicator cell line test is recommended. The test utilizes Vero or 3T6 cells that are inoculated by a >1 mL sample and incubated for 3–5 days at 36 ± 1 °C in a 5% carbon dioxide atmosphere. The cells on cover slips are fixed and stained utilizing Hoechst stain, which is DNA binding. The identification of contaminating mycoplasmas is done by fluorescent microscopy.
The fastest, and probably the most reliable, rapid detection method presently available is the polymerase chain reaction (PCR). Depending on the method — PCR, Q-PCR, RT-PCR or Touchdown PCR — sensitivities and speeds vary. Currently, the fastest and most sensitive method has been presented as Touchdown PCR, which obtains results within 5–8 hours with a sensitivity of 1 cfu/mL (8). The disadvantage of any PCR method is the fact that it cannot distinguish between living or dead cells. This method could result in usable media being rejected for use because of the detection of mycoplasma DNA, even though the cells may no longer be viable. This, however, may be a minor consideration given the broad detection capability, speed and accuracy of the method. At a minimum, it seems that this test is a superb diagnostic for raw materials testing for the purpose of assessing risk and/or a go/no go decision during a cell culture process.
Second Line of Defence: Inactivation
Similar to viral clearance, the best approach to process fluid safety is a multistep, orthogonal inactivation and removal practice. Typical inactivation steps for mycoplasma contaminants are as follows:
antibiotics
heat at 45 °C for 30 min or 60 °C for 10 min
radiation
pH.
As with any inactivation process, these activities can have a detrimental impact on the actual product, particularly regarding the denaturation of proteins. Antibiotics, such as Gentamicin, are often unwanted ingredients in the production process, as these represent the addition of a secondary contaminating substance that must be removed during the purification process. If antibiotics are used, it is critical to ensure that even trace amounts are not present in the final dosage form, because traces could result in anaphylactic reactions in patients.
Heat, pH and radiation all have the common undesirable side-effect of potentially altering media components. Heat is mainly introduced by the use of 80°C water for injection (WFI), into which the powdered media is mixed and solubilized. The mixing process used to achieve a uniform liquid media is commonly sufficient to inactivate mycoplasmas, given the elevated temperature and contact time. Processes that undergo a shift from hot to cold mixing should be evaluated for mycoplasma proliferation, because any possibility of inactivation by heat has been eliminated. Instances have been reported in which temperatures below 80 °C did not effectively eliminate mycoplasma activities. Thus, a heat inactivation step must involve an elevated temperature state, which in itself could have a detrimental effect on the cell culture media or cell expression rate. Radiation has been found to effectively induce a reduction of greater than log 8 viable mycoplasmas at a dose of 25–35 kGy. It is important to note that, at these energy levels, ionizing radiation could also physically inactivate a broad spectrum of potential microbial contaminants, including viruses, bacterial spores and fungi. Tests indicate that doses of ionizing radiation in the range of 25–35 kGy result in a minimal observable effect on media quality. Nevertheless, any inactivation steps require validation to verify the inactivation robustness compared with media product stability and efficacy.
Third Line of Defence: Removal
Mycoplasma removal is most commonly performed by filtrative separation utilizing 0.1 µm-rated membrane filters. As there is no standard challenge test, which would enable comparative 0.1 µm performance evaluation, such filters demonstrate significant variability in the retention of mycoplasmas. Although some have suggested that the use of 0.1 µm filters is uniformly successful in the retention of mycoplasmas (9), the preponderance of evidence suggests that there is no filtration approach that can be considered absolute in the removal of mycoplasmas. In this case, the nomination of “absolute” filter was given to a 0.1 µm-rated filter type that had a titre reduction of greater than log 10 per filter cartridge, representing a challenge level of approximately log 6 per cm2. This would mean every 0.2 µm-rated filter, bacteria challenge tested according to ASTM F 838-05, should also be considered as an “absolute” filter, which is known not to be the case.
The influence of process parameters, fluid volume filtered and organism species determines the retentivity of the filter. The variability of these factors makes it necessary to perform process validation, including product and process parameter challenge tests (10,11). Studies showed that 0.1 µm-rated membrane filters that were able to retain challenges of greater than log 7 per cm2 of Acholeplasma laidlawii were unable to reach the same level of retentivity with Mycoplasma orale or M. pneumoniae (12). Other examples of the variability of the retention capabilities of different filters showed that some filter vendors only guarantee proper reduction levels at specific, higher Bubble Point values (Table 1). Adsorptive retention is effective with some membrane polymers (Figure 1), but can cause unnecessary fouling, such as premature blockage. Furthermore, because adsorptive retentivity is affected by a multitude of parameters, such as temperature, it is a fairly unreliable requisite of filtrative retention.
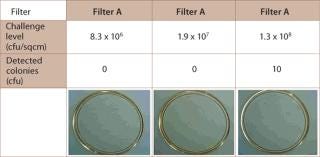
Figure 1: ()
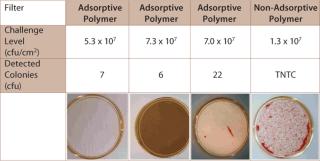
Figure 2: ()
Table 1: Retention dependency on Bubble Point integrity test (Source: Millipore).
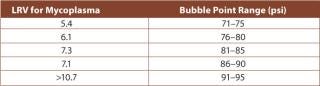
Table 1: Retention dependency on Bubble Point integrity test (Source: Millipore). ()
Differential pressure during filtration has an effect on mycoplasma retention by membrane filtration. Generally, a higher differential pressure can reduce the ability of a filter process to retain mycoplasmas. As mollicutes do not poses a rigid cell wall, higher differential pressures during filtration could cause the organisms to be channelled through the torturous path of the filter’s pore structure.
Other parameters that influence retention efficiency are fluid viscosity — because of the potential drag of the fluid — and flow rates. It is important to note that higher flow rates reduce contact time and, therefore, affect the capture potential, membrane thickness and processing times. All of these parameters play a combined role with respect to the retentivity of the filter. Moreover, 0.1 µm labelled filters are incomparable because, unlike the situation with 0.2 µm filters, there is no standard test for retentivity. A PDA Mycoplasma Task Force is working on a standard challenge test proposal, which could provide recommendations for end-user testing of the performance of 0.1 µm filters. However, at present, it is not known when recommendations for the testing parameters for 0.1 µm filters will be available.
A standard challenge will create comparability, but will not obviate the need for the thorough process validation of mycoplasma-retentive filters. There are a significant number of described retention influencers that must be evaluated to prove filter effectiveness under actual use conditions with the process fluid stream. Laboratory challenge testing can only serve as an indicator that the 0.1 µm-rated filter is a possible proper choice, but filter effectiveness must still be confirmed by product challenges conducted in concert with the process validation program. This approach, already being used with 0.2 µm-rated and viral removal filters, will provide assurance that the chosen filter is appropriate for the specific application. Redundancy or pure reliance on filter manufacturer’s data will not suffice.
Conclusion
The switch from animal-originated media to plant peptone-derived media caused a re-emergence of mycoplasma contamination in cell culture media. This resurgence has resulted in a major problem, particularly within the biotech industry, which relies heavily on large volumes of cell culture media for its mammalian culture processes. Mycoplasmal contamination carried into the process by the raw material source can potentially affect the quality of final product if not treated appropriately. This paper discussed multiple preventive measures to identify and eliminate contamination at various steps, with the preparation of media ingredients and from the media product itself. A single preventive action will probably not be sufficient to ensure a contaminant-free medium; however, an orthogonal, multistep approach can effectively eliminate potential contamination risks. Appropriate risk abatement procedures should be implemented by both the raw material supplier and by the end-user. The burden has to be shared and should not be rest only upon the shoulders of the end-user. Modern detection, inactivation and removal methodologies, supported by process validation, are available options to prevent mycoplasmal contamination and the costly loss of production materials in which they are known — from experience — to result.
REFERENCES
1.) Meltzer, TH, MW Jornitz, and MW. Mittleman. 1997.Surrogate Solution Attributes and Use Conditions: Effects on Bacterial Cell Size and Surface Charge Relevant to Filter Validation Studies, PDA’s Second Asian Symposium, Osaka.
2.) Meltzer, TH, MW Jornitz, and AM. Trotter. 1998. Application-Directed Selection of 0.1 µm or 0.2 µm Rated Sterilizing Filters. Pharm. Technol. 22:116-122.
3.) Jornitz, MW, and TH. Meltzer. 2000. “Addressing Uncertainties in Sterile Filtrations; Substituting Double Filters and 0.1 for 0.2 µm Rating. Pharm. Technol. Eur. 12:20-24.
4.) Lindenblatt, J, MW Jornitz, and TH. Meltzer. 2002. Filter Pore Size Versus Process Validation — A Necessary Debate?”. Eur. J. Parenteral Sci. 7:67-71.
5.) Chizhikov, V. 2008.Mycoplasma Contamination of Biologics: Regulatory Aspects of the Mycoplasma Testing. FDA Workshop: Rapid Methods for Detecting Mycoplasma Contamination, Gaithersburg.
6.) Ikonomi, P. 2008.Effects of Mycoplasma Infection in Cell Culture Systems and development of a PCR-Based Assay for Detection. FDA Workshop: Rapid Methods for Detecting Mycoplasma Contamination, Gaithersburg.
7.) FDA 1993.Points to Consider (PTC) in the Characterization of Cell Lines Used to Produce Biologicals, Dockets Management Branch, Department of Health and Human Services, Rockville.
8.) Potts, B. 2008.Development and Validation of a Touchdown PCR Method for the Detection of Mycoplasma. FDA Workshop: Rapid Methods for Detecting Mycoplasma Contamination, Gaithersburg.
9.) Martin, J. 2007.Qualification of 0.1 µm Filters for Mycoplasma Removal, PDA, Bethesda:163-188.
10.) Carter, J R, and RV. Levy. 1998.Chapter 18: Microbial Retention Testing in the Validation of Sterilizing FiltrationFiltration in the Biopharmaceutical Industry, Marcel Dekker, New York.
11.) Akers, JE. 2008.Chapter 6: Microbiological Considerations in the Selection and Validation of Filter Sterilization. Filtration and Purification in the Biopharmaceutical Industry, informa, New York.
12.) Jornitz, MW, and H. Bromm. 2007.Performance Comparison of “0.1 µm” Rated Filters Using Mycoplasma Challenges, PDA, Bethesda:147-163.
13.) Meltzer, TH, and MW. Jornitz. 2006. Pharmaceutical Filtration – The Management of Organism Removal, PDA, Bethesda.
14.) Meltzer, TH, and J. Lindenblatt. 2002. Selecting 0.1 or 0.2/0.22-Rated Filters, Consideration of Rates of Flow. Eur. J. Parenteral Sci. 7:111-114.
15.) Mittlemann, MW. 2001. Filter Validation: Bacterial Hydrophobicity, Adsorptive Sequestration and Cell Size Alteration. PDA J. Sci. Technol. 55:422-428.
You May Also Like