Spray-Dry Manufacture of Vaccine Formulations
Development of vaccines and immunotherapeutics has expanded rapidly due to technological advances in the fields of molecular biology and bioprocess engineering, as well as a smoothing of management and material logistics worldwide. Expression platforms and novel cell lines have enabled creation of increasingly complex vaccines. The advancement of vaccine formulation development is also capitalizing on new advances in manufacturing that use model-based methodologies gleaned from physiochemical principles, process analytical tools, and systematic approaches to problem solving. Herein we highlight recent advancements in spray-dried vaccine formulation, which has been successfully applied to develop stable and effective formulations across a spectrum of vaccine types.
Vaccine development has been incorporated into the era of biotechnology. The field of vaccines is as old as small-molecule therapeutics, with Jenner’s smallpox vaccinations in 1796 and Withering’s dosing of digitalis in 1785. Novel approaches to immunotherapies range from gene-based vaccines (1,2) to protein subunits (3) to protein complexes such as enveloped and nonenveloped virus-like particles (4,5) and cell based therapies (6). As vaccines have increased in number and quality while varying in type, novel adjuvants have been advanced that focus on targeting specific cellular targets and immune responses (7,8).
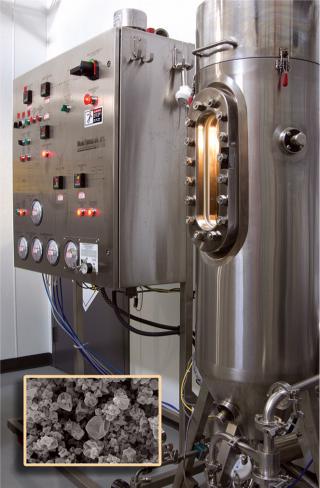
Spray dryer at Bend Research, Inc. (inset: spray-dried particles) ()
Alternative and enabling methods of vaccine formulation are also being explored. Formulation research has focused on creating and defining value-added technologies to solve problems in solid-state formulations that are not tractable using classic dry-state manufacturing technologies. Technologies such as spray drying (9,10,11), spray freeze-drying (12), and foam drying (13,14) are being evaluated for applications in biotherapeutic and vaccine formulations.
Clinical and commercial utility is dictated by the ability to develop robust, scalable, and reproducible manufacturing processes. For scaled-up applications, it is imperative that understanding and application of engineering, chemistry, and biochemistry fundamentals are used to develop formulation and process models (15). On-line and off-line process analytical technologies (PATs) may be used to quantitatively define the effects of unit operations on desired product specifications. Below we present a methodology for creating a robust spray-dried vaccine as well as process steps to consider in defining and controlling a spray-dry R&D and manufacturing process.
Current State and Drivers for Value-Added Technologies
Formerly a relatively staid field, vaccine development continues to increase in importance to the pharmaceutical industry. It is well established that biopharmaceuticals represent an increasing percentage of the pipelines and portfolios for large pharmaceutical companies, either through organic growth or acquisitions. Demand for vaccines is expected to grow from US$11 billion to $16 billion by 2015, with a predicted annual growth rate for vaccines in the U.S. of 6.7% (16). That growth is contingent on advancing the identification of novel disease targets aswell as production, formulation, and delivery strategies.
New vaccines are being developed to treat illnesses in both developed and developing nations. In addition to cancer, infections targeted for immunotherapy include influenza, pneumonia, Staphylococcus aureus, human immunodeficiency virus (HIV), rotovirus, malaria, and shigella. Effective application of vaccines in countries such as the United States as well as in developing countries will largely require novel development, manufacturing, and logistical technologies. Those include new vaccine targets, novel mammalian and nonmammalian cell-based expression systems, PATs, and delivery mechanisms. Formulation technologies can be used to address challenges in shipping and distribution logistics as well as patient delivery. Vaccine storage and delivery technologies are being developed for specific use in developing nations, where hurdles to clinical applications exist.
Logistical and formulation-based stability issues can be solved through appropriate application of dry-powder technologies. Thermostable dry-powder formulations can mitigate the need for cold-chain storage and increase effective distribution of vaccines in both developed and developing countries. Dry-powder– formulated vaccines can stabilize antigens for months at temperature exposures up to 50°C. As Figure 1 highlights, solid-state analytical tools such as modulating differential scanning calorimetry (mDSC) can be used to help define powder characteristics. Engineered particles can also enable patient delivery using noninvasive routes of administration such as intranasal passages and inhalation delivery to the pulmonary airspace.
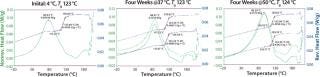
Figure 1: ()
Goals of a Spray-Dried Formulation
Vaccine Antigen Stabilization: Develop a formulation that will support salt and hydrogen bonding to stabilize the protein antigen in the correct tertiary structure.
Powder Stabilization: Powder properties should be amenable to the long-term stability of an antigen, be free flowing, and handle well for downstream process requirements.
Particle Characteristics/Delivery: A spray-dried powder commonly will be resuspended just before parenteral delivery, which cannot allow formation of protein or excipient aggregates. New routes of delivery being explored for vaccine delivery (such as pulmonary and intranasal delivery) depend on particle characteristics and aerosol potential.
Activity: In vitro and in vivo potency and efficacy should be assayed during formulation feasibility and during long-term stability studies.
The clinical availability of multivalent vaccines — e.g., Prevnar (Pfizer) and Gardasil (Merck) — has obviated the fact that major research, development, manufacturing, and logistical efforts are currently under way to increase the breadth of vaccines. Large pharmaceutical companies and nonprofit organizations — e.g., PATH and the Bill and Melinda Gates Foundat
ion — have current research efforts focused on delivering new vaccines to clinics in both developed and developing nations. Combine those research and clinical application efforts with the rise of manufacturing in countries such as China and India, and the scope of the worldwide vaccine effort quickly becomes apparent.
Methodology
Spray-dry formulation and manufacturing is one tool in the vaccine supply chain arsenal that has not been thoroughly applied. In collaboration with development partners, we are seeking to define an application space for spray drying in vaccine development. The gentle physical aspects of this technology are amenable to manufacturing an array of stable and clinically advanceable vaccines.
Use of spray drying to address formulation and stability concerns for dry-state vaccines requires an understanding of critical performance attributes for a desired formulation. Evaluation of key vaccine and adjuvant properties as well as formulation and process guidance maps based on physicochemical excipient and vaccine properties streamline formulation screening to enable lead formulation selection. The tunable and robust nature of spray drying, along with its rapid solvent evaporation kinetics, lends itself to the manufacture of spray-dried biologics, including vaccines (17).
The goal of spray drying a formulation containing a biopharmaceutical agent is to trap the vaccine antigen and adjuvants (for example) inside an amorphous excipient matrix, thus stabilizing them in a dry state. Excipients should limit antigen mobility and thus decrease the potential for failure modes such as aggregation. Salt bridges and hydrogen bonds are known to stabilize proteins in an aqueous state, and it may be important to preserve such interactions in the dry state as well. This approach has been fundamental to dry-state formulation technologies other than spray drying (e.g., lyophilization). Introduction of excipients such as polymers with high glass-transition temperatures (Tg) may also facilitate development of a vaccine-stabilizing spray-dried formulation. High-Tg “skinning” (surface-acting) polymers can also affect the surface properties of spray-dried particles.
The first discussion topic in considering spray-dry manufacture of a vaccine formulation is often thermal exposure. A spray-dry process uses drying gas to rapidly evaporate solvent from atomized particles, leaving behind only the solid components of a solution. Spray drying is a thermally “soft” process because material spends only a short time in the spray dryer. Because of evaporative cooling during the drying process, temperatures experienced are at or below the outlet temperatures. Wet bulb and outlet temperatures define the thermal gradient experienced by spray-dried particles (Figure 2).
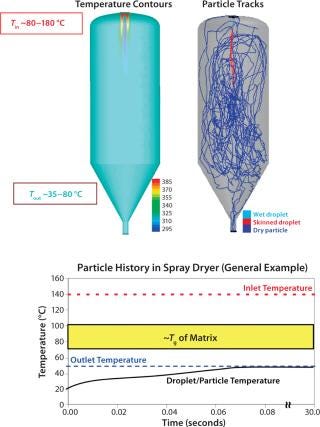
Figure 2: ()
Thermal stress can affect tertiary structure and induce amino-acid modifications, so vaccine potency and activity can be changed by heat. Thermodynamic instability and water loss play a role in protein stress induced during exposure to elevated temperatures. Temperature susceptibility for vaccines in solution depends on a number of factors such as vaccine type, buffers, low– molecular-weight excipients (e.g., sugars), and high–molecular-weight excipients (e.g., polymers). Temperature susceptibility in the solid state is largely defined by vaccine type, but stability is greatly influenced by excipient thermal mobility, which is tied to excipients and water content in a formulation (Table 1).
Table 1:
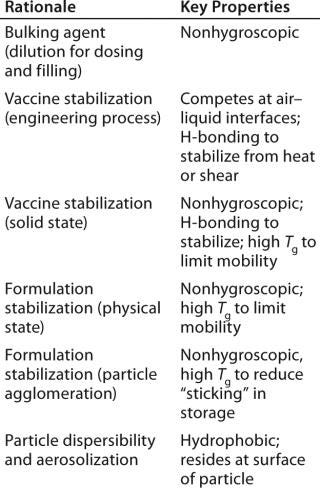
Table 1: 194; ()
Spray-Drying Features
Mild Temperature Exposure:
Short residence time (<30 seconds) in the drying chamber
Evaporative cooling (~Twet bulb)
Product exposure (~Tout)
Product exposure (< < Tin)
Fast Drying (solidification in milliseconds)
Tunable Product Properties:
Optimize with drying conditions and atomization
Powder amenable to a number of delivery routes
Commercial Viability:
Acceptable cost of goods
Continuous, scalable
High product recoveries
Defining ideal formulation, stability, and delivery goals for a vaccine product (based on potency and target dose) is important for providing a rational approach to achieving desired formulation performance attributes. The “Goals” box lists typical goals of a spray-dried biotherapeutic/vaccine formulation.
Formulation and spray-dry process flows have been published and are routinely used to streamline formulation and spray-dry process decision-making to achieve desired formulation attributes (18). The information presented in such guidance maps has been accrued by using historic rational and empirical formulation approaches to solve key challenges for biotherapeutic compounds. A mechanistic understanding of excipient characteristics under anticipated stability and point-of-care conditions is critical to predicting formulation stability and performance.
Careful spray-dry process development rooted in engineering fundamentals combines with rational formulation guidance to manufacture a powder formulation that exhibits desired critical quality attributes (CQAs) within the quality-by-design (QbD) paradigm. Quality attributes for parenteral delivery are often described as “a powder that solubilizes quickly, without aggregation, and that maintains product efficacy during storage.” Aerosol powders often have D10, D50 and D90 particle-distribution specifications; they may havefine-particle fraction and emitted dose criteria as well. Combining careful formulation considerations and spray-dry process development with a focus on delivery of a clinically viable product can lead to a high-performance biotherapeutic formulation that can be manufactured using a scalable process (Figure 3).
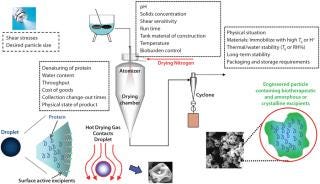
Figure 3: ()
The spray-drying process manufacture of dry-powder vaccine formulations is widely applicable to early stage discovery programs and advanced formulation development stages and to commercialization. Small-scale proof-of-concept programs can make powder at the 200-mg to 300-mg scale, whereas large manufacturing-scale spray driers can produce metric tons of powder. Figure 4 highlights equipment scales used during different stages of a development and manufacturing program.
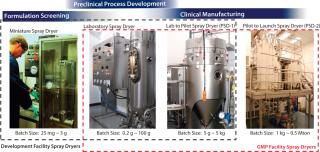
Figure 4: ()
Considerations in Spray-Dry Optimization
The end goal of a rationally designed spray-dry process is a well understood methodology that can reproducibly manufacture high-quality powder and translate to different spray-dry scales. Process impacts on critical formulation attributes must be considered during formulation development. Steps included in a spray-dry manufacturing process can be broken down into a few basic unit operations, which include solution preparation, atomization, drying, collection, characterization, and stability testing.
Solution preparation is an often-overlooked and critical aspect of spray-dry processes. A vaccine must be stable in solution before the formulation is converted to a dry powder. Depending on the scale of the manufacturing effort, solution stability demands may be on the order of minutes or exceed 24 hours. Refrigerated glass-lined tanks, filtration, shear minimization, and buffer considerations should be taken into account for solution stability in the preparation step.
Atomization: The impact of atomization on a vaccine is an important consideration because shear exposure is a concern for product integrity. Nozzle type not only affects shear exposure on a vaccine, but it also determines particle size, which can influence particle dissolution rates and surface exposure of the dried vaccine as well as downstream handling for fill–finish process steps. A range of nozzles is available to select from for spray drying, and proper consideration should be taken for anticipated vaccine sensitivity to shear and desired product profile. Off-line nozzle tools have been built around droplet characterization, which also can be determined by solution properties dictated by excipients to match or optimize droplet diameters across scales.
Drying Chamber: Appropriate removal of water through the exposure of drying gases is critical to maintaining vaccine integrity. The physical stability of particles is maintained during the spray-drying process through the rapid drying kinetics of high–surface-area droplets. Rapid quenching of those droplets to a low-mobility state through use of high-Tg excipients is critical to achieving homogeneous amorphous dispersions. Because spray-dried powders are formulated such that their physical stability depends on molecular mobility rather than thermodynamic miscibility of the components, phase-appropriate tools can be selected during formulation optimization to ensure that a robust formulation and process are selected (18).
Collection: Development-stage biotherapeutics are often high-value products relative to small molecules. So a relatively high manufacturing yield is critical to acquiring enough powder for analytical testing. Spray drying uses cyclones for powder collection, and varying models can be used to define optimal geometry for collecting a desired particle size. Particles can range from 2 µm for inhalation to 30 µm for nasal or parenteral delivery. Ensuring proper collection efficiency across the desired range is critical to feasibility studies and scale-up — both for good laboratory practice (GLP) testing and current good manufacturing practice (CGMP) manufacture.
Stability: It is important to consider what failure mechanisms may be encountered during manufacture and storage of a spray-dried vaccine formulation. Anticipated mechanisms of failure and data generated during early feasibility studies help define formulation needs regarding vaccine stabilization or particle stabilization. Tg as a function of anticipated storage conditions should be evaluated.
Characterization of spray-dried formulations usually involves three performance aspects of the dry-powder vaccine: the solid state, solution in vitro assays, and in vivo efficacy. Proper selection of a suite of analytical tools is important, as is designing a potential action plan around detection of anticipated failure mechanisms. As with any other modern development program,a rationally designed characterization protocol should be defined early on based on critical quality attributes and desired product specifications. Analytical tools should assay antigen integrity and potency as well as excipient state.
Formulation Characterization
The goal of formulation characterization is to determine the integrity of a vaccine antigen and the state of the matrix and performance-enhancing excipients in its formulation. The role and utility of a selected suite of analytical tools should be defined before onset of a characterization program, with known/anticipated mechanisms of failure identified as well as desired product characteristics. For vaccine formulations, a range of solid-state characterization tools and solution-state antigen characterization assays is available. During feasibility and long-term stability studies, in vivo efficacy studies may be performed as well.
Solid-state characterization tools include modulated differential scanning calorimetry (mDSC), powder X-ray diffraction (PXRD), water content analysis (usually Karl Fischer titration analysis), and scanning electron microscopy (SEM). Combining those assays provides an intrinsic understanding of the dry-state formulation and provides guidance for next-step processing conditions, stability, and performance.
Solution in vitro assays include analysis of solution potency, vaccine structure, resuspension rates, and turbidity. The outcome of solution DSC and circular dichroism (CD) assays can provide information on protein structure after spray drying and resuspension. Before in vivo testing, in vitro potency tests such as sodium-dodecyl sulfate polyacrylamide gel electrophoresis (SDS-PAGE), size-exclusion or reverse-phase chromatography (SEC, RPC), multiangle light-scattering analysis, and enzyme-linked immunosorbent assays (ELISAs) as well as antigen-specific assays (e.g., the influenza SRID assay) can provide potency information. Turbidity assays during resuspension-rate analysis can indicate loss of vaccine integrity during spray-drying or resuspension issues induced by excipients or antigen concentration. Solution and solid-state results in concert can help create formulation and process guidance maps.
In vivo efficacy studies can be used early in development and during long-term stability studies. Antibody titer assessments after vaccine challenge are routinely performed at this stage. An early in vivo study can be used to determine the robustness of solid-state an
d in vitro analyses for quantifying quality attributes that correlate with in vivo efficacy. And an in vivo study can be used to derisk formulation development early on and also be used as a performance metric during stability studies. To ensure the desired immune response, consider the mechanism of delivery for an in vivo program.
A Modern Alternative
Solid-state formulation and development tools such as spray drying could improve patient access to vaccines and enable novel delivery routes for inducing robust immunogenic responses in target tissues. Clinicians, pharmaceutical companies, and nonprofit organizations are increasingly identifying methods of delivering vaccines to patient populations that are not currently being served (often due to logistical issues with cold-chain storage).
Big pharma, biotech companies, governmental agencies, and nonprofit organizations have access to technologies that were not available toprevious researchers. New technologies should be defined and can be capitalized upon to solve those access problems. Potentially transformative technologies such as spray drying might not only affect vaccine clinical delivery through generation of engineered particles for inhalation aerosols, but also could improve shipping and logistical approaches for vaccine distribution through mitigation of cold-chain storage issues. Novel technologies are accessible, and they should be capitalized upon to improve human lives.
About the Author
Author Details
Corresponding author Jeff Breit is director of inhalation technology, and Devon DuBose is a senior research engineer at Bend Research Inc., 64550 Research Road, Bend, OR 97701; 1-541-382-4100, fax 1-541-382-2713; [email protected]; www.bendresearch.com.
REFERENCES
1.) Srivastava, I. 2003. Gene Vaccines. Ann. Intern. Med. 138:550-559.
2.) 2012..
3.) Iyer, V. 2012. Formulation Development of a Plant-Derived H1N1 Influenza Vaccine Containing Purified Recombinant Hemagglutinin Antigen. Hum. Vaccin. Immunother. 8:453-464.
4.) Swenson, D. 2008. Monovalent Virus- Like Particle Vaccine Protects Guinea Pigs and Nonhuman Primates Against Infection with Multiple Marburg Viruses. Expert Rev. Vaccines 7:417-429.
5.) Richardson, C. 2013. Norovirus Virus- Like Particle Vaccines for the Prevention of Acute Gastroenteritis. Expert Rev. Vaccines 12:155-167.
6.) Lawrence, F. 2001. Dendritic Cell- Based Xenoantigen Vaccination for Prostate Cancer Immunotherapy. J. Immunol. 167:7150-7156.
7.) Corradin, G, and G. del Giudice. 2005. Novel Adjuvants for Vaccines. Curr. Med. Chem. (Anti-Inflammatory and Anti-Allergy Agents) www.researchgate.net/publication/228475853_Novel_Adjuvants_for_Vaccines/file/32bfe5101bb8c1646d.pdf 4.
8.) Visciano, M. 2012. Effects of Adjuvants on IgG Subclasses Elicited by Virus-Like Particles. J. Translat. Med. 10:4.
9.) Ohtake, S. 2010. Heat-Stable Measles Vaccine Produced By Spray Drying. Vaccine 28:1275-1284.
10.) Breit, J, and D. Dubose. 2003..
11.) McAdams, D. 2012. Spray Drying and Vaccine Stabilization. Expert Rev. Vaccines 11:1211-1219.
12.) Maa, Y. 1999. Protein Inhalation Powders: Spray Drying vs Spray Freeze Drying. Pharm Res. 16:249-254.
13.) Jangle, R. 2012. Vacuum Foam Drying: An Alternative to Lyophilization for Biomolecule Preservation. Indian J. Pharm. Sci. 74:91-100.
14.) Pisal, S. 2006. Vacuum Foam Drying for Preservation of LaSota Virus: Effect of Additives. AAPS PharmSciTech 7:E30-E37.
15.) Dobry, D. 2009. A Model-Based Methodology for Spray-Drying Process Development. J. Pharm. Innov. 4:133-142.
16.) Venkataramanan, M. 2011. Biologics Market Predicted to See 6.5% Yearly Growth in Demand, Spurred By New Cancer and Infectious Disease Therapies. Nat. Med. Spoonful of Medicine blog blogs.nature.com/spoonful/2011/10/biologics_market_predicted_to_1.html..
17.) Sadrzadeh, N Solid-State Stability of Spray-Dried Insulin Powder for Inhalation: Chemical Kinetics and Structural Relaxation Modeling of Exubera Above and Below the Glass Transition Temperature. J. Pharm. Sci. 99:3698-3710.
18.) Baumann, J. 2013. Amorphous Dispersion Formulation Development: Phase- Appropriate Integrated Approaches to Optimizing Performance, Manufacturability, Stability, and Dosage Form. Drug Dev. Deliv www.drug-dev.com/Main/Back-Issues/FORMULATION-DEVELOPMENT-Amorphous-Dispersion-Formu-605.aspx..
You May Also Like