Working with a Powerful and Robust Mixed-Mode Resin for Protein PurificationWorking with a Powerful and Robust Mixed-Mode Resin for Protein Purification
May 1, 2011
Orthogonal methods for assuring robust downstream purification are critical to today’s demanding downstream process industry. Regulatory scrutiny on the immunogenic propensity of drugs has increased and broadened over the past two decades. Although immunogenicity can come from a number of sources, common concerns include host-cell proteins and aggregates. Constantly lurking in the background are other issues such as viral clearance, DNA levels, and so on. Those problems can be addressed simultaneously with the mixed-mode chromatographic support known as ceramic hydroxyapatite (e.g., Bio-Rad’s CHT product) or hydroxylapatite. It offers unique selectivities and often separates biomolecules that appear homogeneous using other chromatographic methods. The diverse binding capabilities of ceramic hydroxyapatite for host-cell proteins, leached protein A, antibody dimers and aggregates, nucleic acids, and viruses allow its use at all stages from initial product capture through final polishing. Recent advances in understanding the properties of ceramic hydroxyapatite have allowed for development of elution/processing methods that significantly extend its method robustness at manufacturing scales.
PRODUCT FOCUS: PROTEINS
PROCESS FOCUS: DOWNSTREAM PROCESSING
WHO SHOULD READ: PROCESS DEVELOPMENT AND MANUFACTURING
KEY WORDS: CHROMATOGRAPHY, VIRUS SAFETY, CONTAMINATION, IMMUNOGENI#CITY
LEVEL: INTERMEDIATE
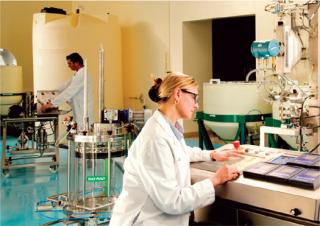
Introduction to Ceramic Hydroxyapatite
Ceramic hydroxyapatite is a mixed-mode resin. Calcium ions on the surface bind to either carboxyl clusters or phosphoryl groups on target molecules in a metal-affinity type of mechanism. Phosphate groups on the surface, alternatively, interact with amines or other positively charged groups on the surface of proteins (or other molecules) in a classical cation-exchange (CEX) mechanism. Because of the unique and well-defined mixed-mode binding of proteins to ceramic hydroxyapatite, the medium possesses separation properties that are unique in the field of chromatography. It is superior to other technologies in its ability to remove aggregates readily (down to below detectable levels in many cases). A typical purification step simultaneously removes 1–5 logs of endotoxin, leached protein A, viruses, nucleic acids, host-cell proteins, and other impurities (1,2,3,4). Used in >15 FDA-approved commercial processes, ceramic hydroxyapatite is used to purify a wide range of protein therapeutics.
CHT binding of basic proteins becomes stronger with decreasing pH because of increasing positive charge on a protein. In general, the lower the pH of a buffer, the stronger is the binding to the support, and the higher is the molarity of eluant required to desorb the protein. This reflects the dominant CEX component of the interaction, but selectivity is distinct from classical cation exchange. Concurrent repulsion of amines by calcium sites and geometric distribution of charges impart a unique stereochemical element that sometimes endows hydroxyapatite chromatography with the ability to discriminate among closely related protein variants. Published examples of this phenomenon include separation of IgG from IgM (5); fractionation of light-chain idiotypes from monoclonal antibody (MAb) mixtures with common heavy chains and fractionation of bifunctional antibodies from complex parent–sibling mixtures (6,7,8,9,10,11,12); separation of minibodies from aggregates (13); and complete resolution of lactate dehydrogenase isozymes using nonceramic hydroxyapatite (14).
CHT Applications
Antibody Purification: With IgGs, ceramic hydroxyapatite is typically used for an intermediate or final polishing step; however, in some cases it has been used for initial capture. Antibodies can be eluted either with phosphate or NaCl, each possessing slightly different desorption properties. One advantage of ceramic hydroxyapatite is that the eluate comes off in relatively high-salt conditions, which makes elution adjustment for a subsequent HIC step relatively easy. By contrast, elution from HIC usually involves low-salt conditions, ideally positioning its eluate for application to ceramic hydroxyapatite with little adjustment. Depending on the exact elution conditions, similar advantageous placements before or after ion-exchange steps have also been reported (1)
Ceramic hydroxyapatite has been used as a primary method for direct capture of IgMs from cell culture supernatants (2). It has also been used to further purify IgAs from Cohn Fraction III (15). As noted, the largest use to date is in MAb purification due to the ability of ceramic hydroxyapatite to remove many or all relevant impurities from their process streams. Table 1 illustrates typical clearances in a CHT step.
Table 1: Typical impurity clearances seen with CHT(4,16)
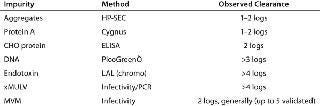
Table 1: Typical impurity clearances seen with CHT(4,16) ()
One of ceramic hydroxyapatite’s most powerful properties is its ability to separate monomeric IgG from dimers and higher-order aggregates. Aggregate removal is especially important here because these species have been implicated in unwanted clinical immunogenic responses (17). Figure 1 shows the ability of ceramic hydroxyapatite to remove aggregates down to a level of detection of <1% from a feedstream containing 40% (by mass) of dimers and aggregates; Figure 2 shows a similar study in which much lower levels of aggregates were equally well removed down to a level of detection (LoD) of 0.03% (18). Finally, it has also been used to separate antibody fragments (Fabs) from Fc fragments (19).
7f7f7;margin-bottom:5px; margin-right:5px; margin-left:5px;margin-top:5px;cursor:pointer;” >
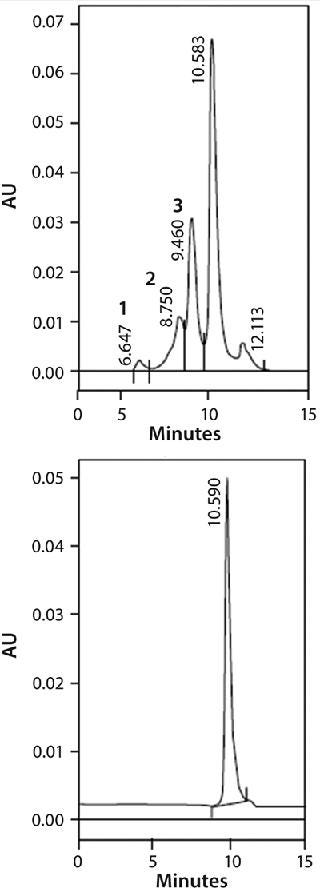
Figure 1: ()
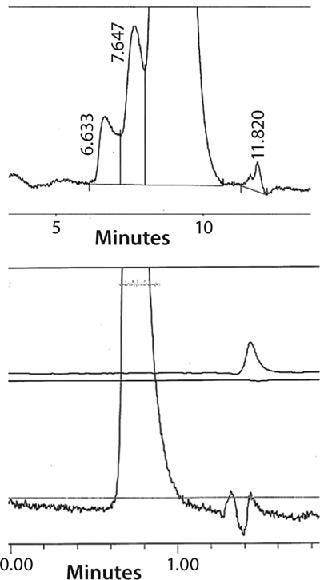
Figure 2: ()
Viruses and Other Vaccine-Related Products: For many years, ceramic hydroxyapatite has been used to purify viral vectors and similar vaccine-related products. The mechanism involved often relies on a high degree of multisite binding between phosphate groups on the surface of the target bioentity and calcium ions on the CHT surface. Both flow-through and bind–elute modes have been used. In this context, ceramic hydroxyapatite has been used in purifying papillomavirus virus-like particles (VLPs) from yeast and other cell types (20); Dengue virus (21); VLPs from bacterial fermentation (22); adenoassociated viruses and adenoviruses (23,24,25); gene therapy vectors from Moloney murine leukemia virus (MoMuLV) (26); and many others (27,28).
Acidic Protein Purification: Owing to the ability of calcium to bind to negatively charged clusters of carboxyl groups, ceramic hydroxyapatite has found a unique place in purifying acidic proteins. Generally, acidic protein binding can be increased by adding small amounts of calcium to a sample load. Elution is carried out by increasing the amount of phosphate in the buffer following a wash step. As expected, acidic proteins are retained more weakly with increasing pH (28). A number of specific examples have been presented for acidic protein purification (29,30,31).
Robust Elution Methods
Ceramic hydroxyapatite binds hydrogen ions on its surface through oxygen atoms attached to the phosphate (32). These ions are in equilibrium with cations in the mobile phase. An increase in cation concentration (during salt elution) releases the protons, leading to a transient pH drop — and the reverse is also true. At pH values <6.5, some CHT dissolution can occur. To overcome this challenge, two strategies have been developed. Each has proven to dramatically extend the robustness of ceramic hydroxyapatite at manufacturing scales.
The first method involves adding a cobuffer (such as 2′-(N-morpholino)ethanesulfonic acid, MES) to the eluant, which minimizes pH excursion during elution. The second strategy involves a simple wash step immediately before elution to convert the acidic surface of ceramic hydroxyapatite to a neutral salt form (33). The wash is extremely gentle, inexpensive, and easy to formulate. It comprises small amounts of a buffer (such as Tris, though a number of others can be used), low levels of NaCl, and 5 mM phosphate, at pH 7.75. Typically, six column volumes of such a solution are sufficient to completely convert the CHT surface. Such conditions can totally eliminate the pH drop during elution (Figure 3).
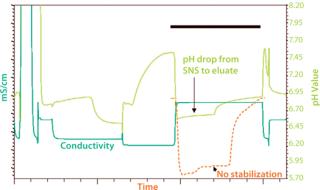
Figure 3: ()
That second technology is known as the surface neutralization system (SNS), for which Bio-Rad has a pending patent. A number of studies have indicated that chromatographic properties of ceramic hydroxyapatite (aggregate removal, host-cell and DNA clearance, binding capacity, and so on) are unaltered with insertion of this step (33). Finally, adding with either method parts-per-million levels of calcium (as calcium chloride) to most buffers used during CHT purification has demonstrated an additive effect on the robustness of this method. These concepts of calcium, cobuffer, and SNS technology are more fully explained in other recent publications (34,35).
About the Author
Author Details
Mark A. Snyder is manager of the process R&D application group at Bio-Rad Laboratories, Inc., 6000 James Watson Drive, Hercules CA 94547; 1-510-741-4675; [email protected]; www.bio-rad.com. CHT is a registered trademark of Bio-Rad Laboratories, Inc.
REFERENCES
1.) Gagnon, P. 2008. Improved Antibody Aggregate Removal By Hydroxyapatite Chromatography in the Presence of Polyethylene Glycol. J. Immunol Meth. 336:222-228.
2.) Hensel,. An Effective Platform for Purification of IgM Monoclonal Antibodies Using Hydroxyapatite.
3.) Franklin, SG. 2002.Technical Bulletin 2849: Protein A Removal from IgG on CHT™ Ceramic Hydroxyapatite Support, Bio-Rad Laboratories, Inc., Hercules.
4.) Gagnon, P. 2006. A Ceramic Hydroxyapatite-Based Purification Platform: Simultaneous Removal of Leached Protein A, Aggregates, DNA and Endotoxins from MAbs. BioProcess Int. 4:50-60.
5.) Brooks, TL., and A. Stevens. 1985. Preparative HPLC Purification of IgG and IgM Monoclonal Antibodies. Am. Lab. 17:54-64.
6.) Bukovsky, J, and RH. Kennett. 1987. Simple and Rapid Purification of Monoclonal Antibodies from Cell Culture Supernatants and Ascites Fluids By Hydroxylapatite Chromatography on Analytical and Preparative Scales. Hybridoma 6:219-228.
7.) Josic, D. 1991. Purification of Monoclonal Antibodies By Hydroxylapatite HPLC and Size-Exclusion HPLC. Biol. Chem. 372:149-156.
8.) Juarez-Salinas, H. 1984. Ultrapurification of Monoclonal Antibodies By High-Performance Hydroxylapatite (HPHT) Chromatography. BioTechniques 2:164-169.
9.) Juarez-Salinas, H. 1986. Separation of IgG Idiotypes By High-Performance Hydroxylapatite Chromatography. Meth. Enzymol. 121:615-622.
10.) Mariani, M. 1989. Purification of Monoclonal Antibodies for In Vivo Use: A Two-Step HPLC Shows Equivalent Performance to Immunoaffinity Chromatography. Biochromatogr. 4:149-155.
11.) Mariani, M. 1989. Is Mouse IgM Purification on Protein A Possible?. Immunol. Today 10:115-116.
12.) Stanker, LH. 1985. One-Step Purification of Mouse Monoclonal
Antibodies from Ascites Fluid By Hydroxylapatite Chromatography. J. Immunol. Meth. 76:157-169.
13.) Gagnon, P. 2010. Minibodies and Multimodal Chromatography Methods. BioProcess Int. 8:26-35.
14.) John, M, and J. Schmidt. 1984. High-Resolution Hydroxyapatite Chromatography of Proteins. Anal. Biochem. 141:466-471.
15.) Leibl,. 1996. Method for the Isolation of Biologically Active Monomeric Immunoglobulin A from Plasma Fraction. J. Chrom. B 678:173-180.
16.) Snyder, MA. 2009. Polyethylene Glycol Enhances Viral Clearance on Ceramic Hydroxyapatite. J. Sep. Sci. 32:4048-4051.
17.) Rosenberg, AS. 2006. Effects of Protein Aggregates: An Immunologic Perspective. AAPS J. 8:E501-E507.
18.) He, X. 2008.Technical Note 5728: Monoclonal Antibody Purification Using UNOsphere SUPrA™ Media, Bio-Rad Laboratories, Inc., Hercules.
19.) Aberin,. Purification and Separation of Fab and Fc Fragments on IgG Monoclonal Antibodies on CHT™ Ceramic Hydroxyapatite.
20.) Cook, III, and C. James. 2003. Process for Purifying Human Papillomavirus Virus-Like Particles.
21.) Kurosawa,. Purification of Dengue Virus Particles Using Ceramic Hydroxyapatite Chromatography.
22.) Richter, S, and S. Topell. 2006. Process for the Preparative Purification of Virus-Like Particles (VLPs).
23.) O’Riordan, C. 2000. Chromatographic Purification of Adeno-Associated Virus (AAV).
24.) Cannon-Carlson, SV. 2008. Method for Purifying Adenoviruses.
25.) Burova, E, and E. Ioffe. 2005. Chromatographic Purification of Recombinant Adenoviral and Adeno-Associated Viral Vectors: Methods and Implications. Gene Ther. 12:S5-S17.
26.) Kuiper,. 2002. Purification of a Functional Gene Therapy Vector Derived from Moloney Murine Leukaemia Virus Using Membrane Filtration and Ceramic Hydroxyapatite Chromatography. Biotechnol. Bioeng. 80:445-453.
27.) Tsuru, S. 1991. Adsorption and Preparation of Human Viruses using Hydroxyapatite Column. Biomed. Mater. Eng. 1:143-147.
28.) Tan, L. 1991. A Novel Process for Preparing an Acellular Pertussis Vaccine Composed of Non-Pyrogenic Toxoids of Pertussis Toxin and Filamentous Hemagglutinin. Mol. Immunol. 28:251-255.
29.) Ogawa, Hiraide 1996. Effect of pH on Gradient Elution of Different Proteins on Two Types of Macro Prep Ceramic Hydroxyapaptite. Am. Lab. 28:31-34.
30.) 2002.Technical Note #2535: From Transgenic Milk Using CHT ™ Ceramic Hydroxyapatite, Bio-Rad Laboratories, Hercules.
31.) Fujisawa, Kuboki 1991. Preferential Adsorption of Dentin and Bone Acidic Proteins on the (100) Face of Hydroxyapatite Crystals. Biochim. Biophys. Acta. 1075:56-60.
32.) Kwon,. 2009. Effect of Salinity on Hydroxyapatite Dissolution Studied by Atomic Force Microscopy. J. Phys. Chem. C 113:3369-3372.
33.) Snyder, Cummings. 2000.A New, Robust Method for Protein Elution from Ceramic Hydroxyapatite Recovery of Biomolecules XIV, Lake Tahoe.
34.) Cummings, L. 2009. Protein Chromatography on Hydroxyapatite Columns. Meth. Enzymol. 463:387-404.
35.) Cummings, L. Hydroxyapatite Chromatography Purification Strategies of Recombinant Proteins, Methods Navigator Cookbook.
You May Also Like