Designing the Ideal Bioreactor with Single-Use Technology
Bioprocessing companies are hoping for a brighter future in biologics manufacturing that will include ever-higher titers of vaccines and therapeutic proteins grown in cell culture. It would also facilitate bioprocess operations without the recurring challenges that stem from process scale-up and human error. Moreover, that future would also comply with increasingly stringent regulatory and current good manufacturing practice (CGMP) requirements while providing better cost controls than we see today. How far away is this future? Perhaps not too far.
Traditional Design No Longer Ideal
For decades, stainless steel, impeller-driven bioreactor systems were the traditional choice for manufacturing biologics. This has been an adequate bioreactor design for delivering acceptable biological performance and meeting regulatory guidance that governs safe and effective use, especially for biologics manufacturing using robust cell lines.
However, manufacture of new biotherapeutics and vaccines exposed a number of significant challenges in the stainless-steel, impeller-driven bioreactor model. Mechanical shear stress caused by high-speed impellers is not a significant problem for bacterial cells or robust mammalian cell lines such as Chinese hamster ovary (CHO) cells. But shear has shown detrimental effects on cell growth and productivity of anchorage-dependent mammalian cell lines such as Vero and Madin-Darby canine kidney (MDCK) cells in microcarrier processes, especially at large scales (1). In addition, the increasing spatial gradients of key environmental conditions such as pH and dissolved gases (O2 and CO2) during process scale-up in traditional impeller-driven vessels (Figure 1) can plague cell-culture performances, even at smaller working volumes (2).
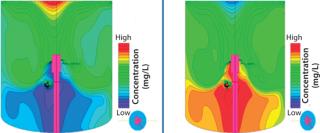
Figure 1: ()
Some cell culture scientists have claimed that the “ideal” future bioreactor was out of reach (3). However, a new wave of innovative, single-use bioreactors under development may be closer to the ideal system. These reactors bypass high capital expense, long installation lead times, and complicated operations associated with stainless-steel systems by eliminating the need for in situ sterilization and cleaning between batches. Thus they open the door to culturing commercial-scale volumes of shear-sensitive cells that are critical to development of new vaccines and biological therapies. As the biotech industry evolves toward new applications such as cell therapies and stem cells, regenerative medicine, and cell-based vaccine production using adherent and shear-sensitive cells, bioreactors will need to provide an ideal environment for them (4).
The Ideal for Cells
For healthy growth, cells — especially mammalian cells — require a hospitable environment that resembles their original in vivo state as closely as possible. In a bioreactor system, all cells should be exposed to similar process conditions to ensure consistency in quantity and quality of the desired recombinant protein (or therapeutic effect) they produce. So it is necessary that their culture environment be as uniform as possible, with minimal spatial gradients across the entire range of bioreactors used during process scale-up.
An ideal bioreactor would thus be capable of fast, homogeneous liquid mixing to minimize effects of spatial gradients in temperature, pH, dissolved oxygen (DO), and nutrient levels in the vessel — especially as its size increases — while providing sufficient particle suspension power to prevent cells from settling on the bottom (5). In traditional bioreactors, mixing times are often improved by increasing agitation rates — sometimes to levels that can be detrimental to certain cell culture processes (6).
High mass-transfer rate capability is also important, particularly for high-density cell cultures, to achieve efficient O2 dissolution into the culture and strip CO2 out. In traditional systems, a relatively high flow rate of large gas bubbles strips CO2 while delivering O2 to a culture, although agitation rate also may be increased while delivering smaller bubbles through a microporous sparger element for improved oxygenation. The challenge here is to maximize mass-transfer rates while delivering large enough gas bubbles to prevent hydrodynamic stresses to cells when the bubbles burst at the top fluid surface (1).
Finally, an ideal bioreactor should impart low shear stress on cells, minimizing mechanical damage from eddies formed by the impeller motion or by bursting gas bubbles. Traditional bioreactors produce increasingly higher shear stress and turbulent kinetic energy dissipation rates during process scale up. But an ideal bioreactor would maintain constant levels of those values despite an increase in working volume, which would improve the robustness of process scale-up.
The Ideal for Users
Biomanufacturers are facing unprecedented pressures from a number of sources. Regulators are increasingly concerned about product safety for patients, and problems in manufacturing processing can compromise that. Meanwhile, biologics manufacturers are under pressure to hold down costs. Although a bioreactor design cannot resolve all bioprocessing cost issues, it is part of an important unit operation for streamlining processes and ultimately providing cost-effective products to the market.
Traditional stainless steel bioreactors require high capital investment to install (even if they are the most available technology), and they are expensive to operate. Single-use bioreactors, on the other hand, offer more economical bioprocessing solutions with greater flexibility in manufacturing capacity. Their capital and operating costs are lowered by eliminating the cleaning and validation requirement between batches of traditional systems, as well as offering easier operations with simpler set-up in a smaller footprint on a factory floor.
An ideal bioreactor would be easy to install, use, and maintain. It would provide an intuitive user interface and be robust to reliably achieve critical process conditions. Remote access to control data through a secured Internet connection would increase operating efficiency and flexibility. Although such a bioreactor could operate using its own controller, it also would be linkable to a facility’s distributed control system (DCS) network, data historian, and other ancillary laboratory equipment such as cell counters and metabolite analyzers.
Disposable bag assemblies should be robust and easy to install in their housings and readily available for design customization (e.g., liquid-handling line configuration for ease of connectivity to upstream processing equipment such as media bags and inoculum containers and downstream processing equipment). The bioreactor system also should be compatible with various types of sensors that users apply for process monitoring.
As with other bioprocesses, regulatory compliance is crucial for bioreactors. To meet CGMP requirements, all materials that contact the product (vessel interior walls, in-line sensors, mi
xing elements, gas sparging, and liquid handling lines) must be manufactured from USP Class VI medical-grade materials. Their suppliers should use validated processes and quality systems for all component manufacturing, with full traceability for changes in raw materials, product design, and manufacturing processes.
Sterilization methods must be validated — whether sterilization-in-place (SIP) for stainless steel systems or gamma irradiation for single-use systems — to ensure a clean, sterile culturing environment to begin with for each use. The US FDA also recommends extractables studies to be performed by suppliers, and leachable studies that may be performed by suppliers and/or users. In addition, a bioreactor control system must meet 21 CFR Part 11 requirements for electronic records and signatures, with documentation of qualification and validation guides available for each bioreactor.
Bioreactor suppliers should work closely with their customers to provide timely technical support and customer service to minimize downtime if a system or part fails. Such companies also should secure a continuous and timely supply chain of their products.
The Future Is Now?
The future may be closing in. We are approaching an era of the “ideal” bioreactor that can better respond not only to the changing environment of biopharmaceutical manufacturing and research, but also to growing cost and regulatory pressures on the biotherapeutics industry. Moving from traditional stirred-tank bioreactor systems toward disposable, single-use technologies is a significant step in that ideal direction. But much yet needs to be done before the future becomes a ubiquitous reality on every factory floor.
About the Author
Author Details
Yas Hashimura is director of application engineering, Daníel Giroux is vice president of research and development, and corresponding author Brian Lee, PhD, is president of PBS Biotech, Inc., 4023 Camino Ranchero, Suite I, Camarillo, CA 93012; 1-805-482-7272, fax 1-805-383-7727; [email protected], www.pbsbiotech.com.
REFERENCES
1.) Chisti, Y. Flickinger, MC 2010.Shear SensitivityEncyclopedia of Industrial Biotechnology, Bioprocess, Bioseparation, and Cell Technology, Wiley, New York.
2.) Parnaik, PR. 2002. Can Imperfections Help to Improve Bioreactor Performance?. Trends Biotechnol. 20:135-137.
3.) Aunins, JG. Flickinger, MC 2010.Viral Vaccine Production in Cell CultureEncyclopedia of Industrial Biotechnology, Bioprocess, Bioseparation, and Cell Technology, Wiley, New York.
4.) Maranga, L. 2002. Production of Core and Virus-Like Particles with Baculovirus-Infected Insect Cells. Adv. Biochem. Eng./Biotechnol. 74:183-204.
5.) Nienow, AW. 2006. Reactor Engineering in Large Scale Animal Cell Culture. Cytotechnol. 50:9-33.
6.) Mollet, M. 2004. Bioprocess Equipment: Characterization on Energy Dissipation Rate and Its Potential to Damage Cells. Biotechnol. Prog. 20:1437-1448.
You May Also Like