Mixing in Small-Scale Single-Use Systems
Despite the advantages of presterile, single-use technologies, mixing is one of their most complex applications. Industry has been progressing toward using single-use bag technologies rather than traditional methods of stainless steel tanks and grades A/B processing because of the positive aspects they impart to end users, including a reduced potential for contaminants, cleaning, sterilization, and capital. These technologies offer simplicity and flexibility (1). However, using them for an operation such as mixing can add processing challenges. For example, solutions in soft-walled bags do not always behave according to typical fluid dynamics as they would in rigid, cylindrical vessels.
Here we review scalability, design considerations, and testing approaches and outline some studies we performed in the early development of small-scale single-use mixing systems for suspensions using the limited technologies currently available.
PRODUCT FOCUS: ALL BIOLOGICS, INCLUDING VACCINES
PROCESS FOCUS: PRODUCTION, FORMULATION
WHO SHOULD READ: PROCESS DEVELOPMENT AND MANUFACTURING
KEYWORDS: SCALE UP, DISPOSABLES, FORMULATION, MEDIA/BUFFER PREPARATION, TEST METHODS
LEVEL: INTERMEDIATE
A Need for Scalable Solutions
A number of companies offer commercially available, well-engineered single-use mixing platforms (e.g., ATMI LifeSciences, Millipore, Thermo Scientific Hyclone, Sartorius Stedim Biotech, GE Healthcare Life Sciences, and Xcellerex). Bag sizes typically range from 20 to 2,000 L, ideal for applications such as buffer and media preparations and for upgrading from older systems of commercially licensed products.
However, the industry needs even smaller-scale disposable mixers. New biotechnology products start in early trial preclinical and clinical phases at small-scale volumes (typically 0.5–20 L). Also, some products such as vaccines can be made at such high concentrations that, in one case, a single 5-L batch can supply thousands of doses (2). Finally, design of industrial-scale bioprocesses should be based on the performance of small-scale prototypes; otherwise, it becomes costly and time-consuming (3).
Finding a good disposable mixing system for a small-scale application is problematic not only because the larger systems are not readily available in a scaled-down form, but also because most available mixing systems on the market require a specific bag film. If other films are already validated by a biotech manufacturer, then implementing a new system with a new bag film becomes difficult. FDA regulations require revalidation when changing from one set of disposables to another (2). Therefore, selection of single-use mixing systems can quickly become limited.
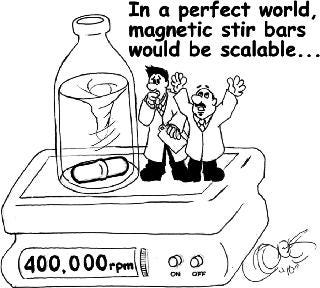
Design Considerations for Small Batches
Preferably, a single mixing unit will be equally suitable for use in a small-scale preclinical environment as in a large-scale production situation (4). Yet, process engineering experts recognize that it is impossible to generalize methods for scaling up all mixing processes (5). Mixer selection depends on specific mixing applications such as blending, stirring, dissolving, or suspending. Other activities to take into account include storage, dispensing, heating/cooling, weighing, and upstream or downstream connections. Ideally, if applicable, all product-contact surfaces of a mixing container assembly and connections should be maintained within a closed system and should have no direct contact with nonsterile equipment components.
Development of single-use mixing systems for stock solutions, intermediates, and final formulation suspensions involve establishment of defined requirements for specialized equipment, appropriate process design, and disposable assemblies configured with necessary ports and other accessories. This matter becomes more complicated for soft-walled disposable, box-like configurations in scale-up mixing applications. For example, square vessels have more turbulent mixing than similar cylindrical tanks as a result of the increased turbulence of eddy currents generated in the corners of the vessel (5). Not only must the container vessel and agitation aspects be scalable, but the tubing, ports, mixing drive, vessel holding apparatus, and platform must be scalable, as required. In addition, bag material and thickness must be taken into account because bags must be crack resistant to fluid movements and maintain integrity with weight load increase. Most existing small-scale options have limitations and require additional manipulations of the bag and processing lines for specific functions.
A 2009 supplier review on disposable mixing systems concluded that bag-to-bag interchangeability from the mixer driver, available room height, start-up times, ease of use, and pricing are important aspects to consider in selection (6). With all this in mind, for early trial small-scale manufacturing, equipment should not be unnecessarily complex or overengineered because it may not be a suitable solution for large scale.
Proper Mixing Controls and Testing
Qualitative and quantitative testing must be performed to develop adequate settings and physical attributes for a given mixing process. These tests can include evaluation of impeller geometry, size, and orientation relative to the vessel (5) as well as revolutions per minute, pitch, mixing time, in- and out-flow, and volume. In addition, a robust and nonvariable mixing system is engineered and tested based on knowledge of a product’s chemical and physical characteristics such as
Fluid viscosity
Solubility
Thermal conductivity of fluid
Fluid density
Particle size and density (7).
A product’s internal reactions, compatibility with bag materials, and additional manufacturing and testing requirements should also be understood. Although some end users make this request, testing a system for integrity before and/or after use is not always feasible in many mixing applications (6). Factors such as stage of manufacturing, integrity, and ensuring minimized particulate generation could be supported by vendor-provided data or end-user data and a risk-based approach.
Depending on the nature of a product, running a qualitative test first can give preliminary indications of efficient mixing as results are achieved immediately, and parameters can be tweaked accordingly during mixing without sampling. Indicators can include absence of visible aggregation, homogeneity, minimal foaming, absence of visible dead pockets in the bags (zones where liquid is present but not circulating), and minimal spattering (when droplets accumulate on the inner sides and/or top of the bag).
Quantitative indicators are variables such as pH or conductivity that achieve steady state as a function of mixing time, for example. For adjuvant suspensions, indicators such as aluminum content, turbidity, pellet weight, adsorption, final product potency and particle
size are appropriate in our experiments because it was the adjuvant-based components that settled out of solution.
Small Mixing Systems Tested
An early phase vaccine formulation contains an aluminum-adjuvant suspension that sediments in a shorter time frame than other common aluminum-based adjuvants. In addition, remixing a viscous adjuvant-based lower layer is difficult once settling has occurred (8). During a first attempt of the referenced process, the product was aggregate-prone. Sedimentation and unmixed regions in the corners of the bag were also observed. A series of evaluations followed to attempt to attain an efficient small-scale mixing system, adequate yield and suspension with volume changes, and a scalable system.
Single-use assemblies used in the following studies consisted of a bag, with a common film type, connected to a closed system manifold provided by the bag manufacturer pre-assembled, overwrap-sealed, and gamma irradiated.
An Efficient Small-Scale Mixing System: Our team chose four different noninvasive single-use systems to mix aluminum-based solutions in two-dimensional bags (Figure 1). The Wave 20/50 EH Electric Wave Mixer system with Touchpanel mixes solutions in disposable bags using a noninvasive rocking wave motion designed to reduce shear forces, product damage, and foaming while sweeping and dispersing solids into the liquid (9). We tested a recirculation line (also known as a pump-around loop): Two tubing lines coming from the bag were aseptically connected and looped through a peristaltic pump to keep the line in circulation. We also tested a rotating drum (used primarily as a lab tissue-culture rotator) by affixing a bag to the drum using cable ties to try another approach without the use of impellers. Bags were rotated 360° to resuspend product. In addition, we tested an external magnetic stir plate with bags containing an internal stir bar.

Figure 1: ()
We added blue dextran to bind to the aluminum-based ingredient in the suspension to identify phase separation. Minimum volumes selected were based on the minimum amount of product to be mixed in the size of container. Maximum volumes selected were based on the Wave Mixer system requiring a ≤75% maximum bag fill-volume for optimal mixing. Bag contents were permitted to settle for a defined period to ensure that a clear supernatant layer was separated by a cloudy blue layer. Most mixing systems will mix within very short times and are tested accordingly; however, for these products, certain necessary ingredient interactions led to longer mixing times. In addition, suboptimal settings can lead to sedimentation that may not be detectable following a shorter mixing period. For these initial comparison tests, the mixing time was 30 min. Both 1-L and 5-L bags were tested, where feasible. As controls, 2-L and 5-L bottles on stir plates were used because of their cylindrical and rigid nature.
The study was designed to provide a basic understanding of each system’s mixing principles and handling (setup, sampling, and limitations) (Table 1). However, all systems require further optimization for best application. At low volumes, the 5-L bags in the Wave Mixer system, recirculation line, and stir plate set-ups had unused areas where the fluid did not travel. To prevent that, we affixed a large, noninvasive clamp to the bag to squeeze the liquid to a common area, leaving the rest of the bag empty.
Table 1: Summarized qualitative mixing efficiency results for various systems tested with single-use disposable bags; WAVE Mixer system produced best results for both 1-L and 5-L two-dimensional bags with mineral-based suspensions at low and high volumes. A√ was given if all volumes tested achieved a successful outcome; an X was given if only one or both volumes tested did not achieve a successful outcome.
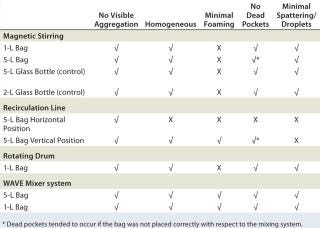
Table 1: Summarized qualitative mixing efficiency results for various systems tested with single-use disposable bags; WAVE Mixer system produced best results for both 1-L and 5-L two-dimensional bags with mineral-based suspensions at low and high volumes. A√ was given if all volumes tested achieved a successful outcome; an X was given if only one or both volumes tested did not achieve a successful outcome. ()
The rotating drum created the most foaming (even without a surfactant), and the recirculation line was difficult to set up to ensure that all parts of the bag had sufficient circulation. The most promising mixing system solution was the Wave Mixer system followed by the stir plate.
Adequate Yield and Suspension with Volume Changes: Keeping mixing settings constant while yielding the same results would reduce validation during scale-up (4). This is not always feasible or practical because activities such as working-volume changes can affect mixing efficiency when blending or dispensing. As the volume increases in the mixing vessel, the flow paths for circulation are lengthened, so the fluid velocity must be increased in proportion to volume if the mixing time is to remain constant (3). For example, we tested several volumes to determine the appropriate settings for the Wave Mixer system. When we applied low-volume settings to high-volume conditions, the product sedimented out, and a higher rpm was required to increase agitation (Table 2).
Table 2: Best conditions mixing a suspension in a 5-L bag using a WAVE Mixer system; settings change based on volume changes and whether air has been evacuated from the bag.
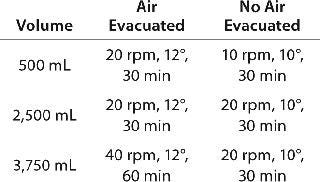
Table 2: Best conditions mixing a suspension in a 5-L bag using a WAVE Mixer system; settings change based on volume changes and whether air has been evacuated from the bag. ()
As part of our final blending processes, we needed to maintain a suspension in the bag while moving it to another part of t
he assembly or directly to the final container (e.g., a vial or syringe). Air gaps or bubbles must be prevented in the line with metered dispensing through a pump (a pressurized pump with a disposable processing line was unavailable at the time we wrote this), which limits the mixing system options. No system tested could both mix and dispense effectively without additional manipulations, high product loss, and/or lack of control. We evacuated air from the bag to attempt dispensing while mixing on the Wave Mixer system. Doing so led to an awkward manipulation and, as can be seen from Table 2, longer mixing times and increased agitation settings.
An outfeed dipping line or bottom drain on a bag would prevent air from displacing liquid in the processing lines, eliminate unnecessary manipulations and maximize product yield. So we requested other mixing bag solutions from the manufacturers. One option was a three-dimensional 3-L bag in which the manufacturer added a stir bar and dip line in the bag such that the entire assembly was provided presterilized (Photo 1). Different volumes and speeds were tested using a stir plate. For proof of concept, we measured the pH in the bag as a function of blending time after we added 6 N HCl solution to a 6 mM NaOH solution. Initial mixing studies showed that blending times of such nonsuspension-based solutions are
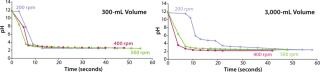
Figure 2: ()
The next step was to test the three-dimensional bag with suspension solutions. Three different quantitative methods showing mixing efficiency of various adjuvant-based suspensions are shown in Figure 4 and 5. These tests all used the mixing bags with stir bar and stir plate, and all show promising results. Samples taken for aluminum concentration were analyzed for elemental aluminum by inductively coupled plasma atomic emission spectrometry (Figure 3). Samples taken for pellet weight were measured after centrifugation and supernatant removal (Figure 4). Samples for turbidity were measured with a Hach portable turbidity meter (Figure 5). Representative results were found only when the bottom sample line was purged before the samples were taken likely due to settling in the line. As testing progressed, we observed that lower mixing volumes (200 mL) could be used. All results show that at both low and high volumes for various mixing times, efficient mixing can be achieved.
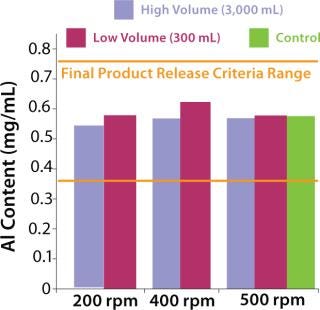
Figure 3: ()
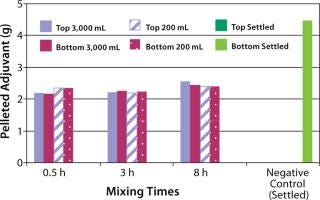
Figure 4: ()
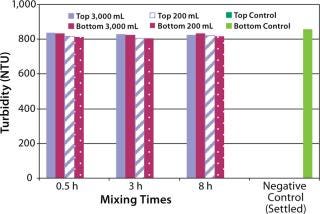
Figure 5: ()
Photo 1:
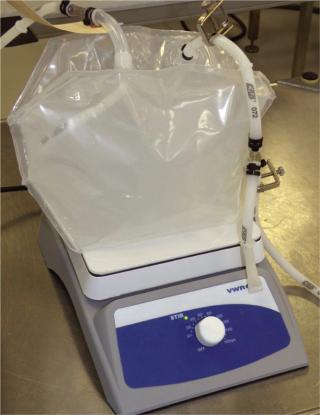
Photo 1: ()
A Scalable System: The three-dimensional bag with stir bar performed well but has one major limitation: It is not scalable (a giant stir bar?). Fortunately, some disposable mixing companies have very recently been developing new small-scale (5- and 10-L) technologies in which the impeller is located inside the bag with both inlet and drain lines and presterilized as part of the bag assembly (Figure 6). These systems already come platform-ready in larger scale. We have had the opportunity to test some prototypes and found that initial results are promising in terms of mixing efficiency.
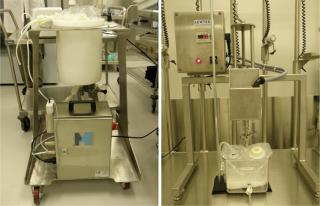
Figure 6: ()
Nevertheless, for new small-scale disposable mixing systems, we need to continue to pay close attention to:
sufficient mixing while maximizing product yield at reduced volumes
validation of supplier-specific films and bag assemblies
equipment validation data
secure coupling/decoupling bags to/from a drive motor connection.
An Innovation Challenge
Small-capacity, low-capital mixing technologies need further advancements for biopharmaceutical processing applications and need to be used with a variety of (or ideally, standardized) bag films. In addition, robust and cost-effective testing methodologies should be promoted to e
nsure adequate product quality and control over the product lifecycle. Although single-use technologies offer several advantages over conventional processing methodologies, the industry currently has a significant unmet need for scalable systems applicable to high-value, low-volume biopharmaceuticals such has monoclonal antibodies and vaccines. Furthermore, mixing systems appropriate not just to pure solutions, but that address challenges intrinsic to suspension-based products are not currently optimized and may represent a market opportunity for innovation. This will be demanding perhaps, but necessary.
About the Author
Author Details
Kirsten Strahlendorf is a process development scientist at Sanofi Pasteur, [email protected]. Kevin Harper is a platform director at Sanofi Pasteur, [email protected].
REFERENCES
1.) Phillips, CW. 2008. It’s Not Whether, but Rather What and How to Implement. BioProcess Int. 6:S6-S9.
2.) Brown, AS. 2006. Disposable Equipment Makes Lasting Gains. ChemicalProcessing.com.
3.) Doran, PM. 1995.Bioprocess Engineering Principles, Academic Press, London.
4.) 2008.Newmix-Levtech Application Note XA011E 0808Rev1Demonstrating Pad-Drive™ Scalability from 25L to 1000L, ATMI, Inc, Minneapolis.
5.) Sandler, HJ, and ET. Luckiewicz. 1987.Practical Process Engineering, a Working Approach to Plant Design, McGraw-Hill, Inc, New York.
6.) Sinclair, A, and M. Monge. 2009. Evaluating Disposable Mixing Systems. BioPharm Int. 7.
7.) Luckiewicz, ET. 2004.Elements of Applied Process Engineering Course Notes, Center for Professional Advancement, New Brunswick.
8.) 2008.Newmix-Levtech Application Note XA002E 0807Rev2Mixing of Aluminum Hydroxide Gel in Water with Pad-Drive, ATMI, Inc, Minneapolis.
9.) Singh, V. 2000.BioProcess Tutorial: Non-Invasive Mixing in Bags — The WAVE Mixer Provides New Options for Sterile Mixing, Wave Biotech, Bedminster.
You May Also Like