Current Issues in Assuring Virological Safety of Biopharmaceuticals
March 1, 2012
The weakest link in the chain is also the strongest. It can break the chain.
— Stanislaw Jerzy Lec, Polish writer, poet and satirist (1906–1966)
Biologicals ushered in a new era for treating debilitating and life-threatening illnesses. According to a Pharmaceutical Research and Manufacturers of America (PhRMA) 2011 report, more than 900 biotech medicines and vaccines are in development that are targeting more than 100 diseases (1). Market researchers expect annual sales of biologics (now at about US$100 billion) to grow two to three times faster than conventional small molecule drugs during the next five years (2). Advances in cell line development, bioreactor design, and purification techniques have led to a sevenfold increase in global protein output from mammalian cell cultures alone from 2000 to 2005 (500 kg versus 3,600 kg) (3).
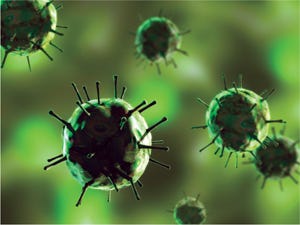
From a virological safety standpoint, biopharmaceuticals have had an excellent safety record during the past quarter of a century (4). This excellent safety profile has not been easy to achieve and can largely be attributed to a viral safety tripod strictly adhered to in the industry: adequate sourcing, documentation of virus clearance evaluation (virus validation studies), and in-process testing (5).
Although it is highly commendable that our current methods of detection and virus safety assurance have provided safe products over the decades, we need to recognize that we are not invulnerable. For example, the industry has had issues related to adventitious agent contamination in recent years of both bulk harvests and production environments (6,7,8,9).
From Prescriptive to Preemptive
During the past few decades, pharmaceutical quality paradigms have experienced a tectonic shift. Traditional approaches were prescriptive and governed by defining set points and operating ranges for process parameters. The current quality paradigm is a risk-based approach that leverages scientific knowledge to select process parameters and unit operations that affect critical quality attributes (CQA). This establishes design space and control strategies that are applicable throughout the lifecycle of a therapeutic.
Initial approaches to ensuring virological safety of biologicals were responsive and disaster-led. Iatrogenic accidents related to vaccine contamination occurred for several reasons: adventitious virus contamination of the production system, incomplete viral inactivation during manufacturing, and residual pathogenicity of the live attenuated virus strains (10). The outcome was incorporation of several industry initiatives to enhance vaccine safety and imposition of stringent regulatory requirements. Current approaches are preemptive and focus on disaster avoidance. The emphasis is on knowledge management rather than simple data accumulation, efficient risk management and an overall lifecycle approach, and continual improvement based on the generation of new information.
Where Are the New Viruses Coming From?
Epidemiological studies strongly suggest that novel infectious agents remain to be discovered. This must be anticipated in public health planning and surveillance programs for emerging infectious diseases. Woolhouse et al. estimate that 10–40 new virus species remain to be discovered by 2020 (11). In addition, some viruses cross the species barrier. For example, the plant circovirus and porcine circovirus have a common ancestor (12). Reportedly, in the past quarter of the 20th century, more than 30 emerging infections were recognized, the majority of which were of zoonotic origin (10). Recombination between viruses is a definite concern. Xenotropic murine-leukemia-virus–related virus (XMRV) is implicated in diseases such as prostate cancer and chronic fatigue syndrome; but there is no conclusive evidence that this is the case (13).
With current globalization as it relates to travel, viruses not indigenous to a particular location can enter new environments if there is a susceptible host population and other factors are conducive. For example, the arbovirus, chikungunya virus (CHIKV) (indigenous in Africa and certain Asian locales) has been introduced into certain areas in Europe and North America in this century with the appropriate insect vector available. CHIKV has revealed extraordinary adaptive capability: It has now repeatedly been associated with a new vector, Aedes albopictus (the “Asian tiger mosquito”) and has dispersed worldwide (14). In addition, xenotransplantation of organs introduces the potential for xenozoonoses (xenosis) (15,16)
Risk and Risk Analysis Tools
Risk is the concept of the day in biotechnology, pushing continuous improvement, better quality management, and greater technology adoption (17). Risk-assessment requires integration of many parameters, including the nature of an extraneous agent, its target species, test limits of detection, the nature and geographical origin of raw materials, manufacturing processes and steps during production, and application of current good manufacturing practices (CGMPs).
Risk evaluation from a virus safety standpoint must include exposures to potential sources of viral burden. That starts with selecting and establishing a master cell bank as well as evaluating manufacturing inputs, downstream purification reagents, and formulation excipients. For example, mammalian antibodies have been used in clonal selection, and animal-derived materials (ADMs) such as porcine trypsin, bovine serum, and animal derived growth factors have been used in manufacturing. Downstream purification reagents include antibody columns for purification. Excipients such as human serum albumin (HSA) were used in the past, and although recombinant HSA is currently used, it does not totally negate the potential for virus burden.
Several methods for risk estimation have been proposed; their utility depends on the task at hand. To maximize benefits from such methods, as with a technique, you should understand their contributions and limitations. For example, once a FMEA (failure modes and effects analysis) identifies potential failure modes and potential outcomes, risk reduction approaches can be applied to eliminate or reduce potential impacts.
The FMEA approach provides a numerical rating system for determining the severity of an event, probability of occurrence, and the ability to detect it. On the basis of those factors, a relative risk (numerical value) is assigned. The risk priority number is a multiple of the relative risk score for each of three variables: severity, occurrence, and detection. This is done for each operating parameter of each process step (18,19).
Risk mitigation plans are an essential component of overall risk management. You should proactively have risk management plans in place. Have corporate virus containment plans in the event of a contamination event with designated teams and corporate- and site-specific documents that delineate responsibilities and actions
. Risk communication is an integral part of risk management. Notifying internal and external agencies should be a part of the risk management strategy.
Sourcing Raw Materials and Supply Chain Management
Raw materials pose a significant area of vulnerability in biopharmaceutical manufacture, especially in view of the wide variety of constituents used, their large volumes, and complex risk profiles. Table 1 lists some issues of concern related to raw materials sourcing.
Table 1: Challenges related to raw materials
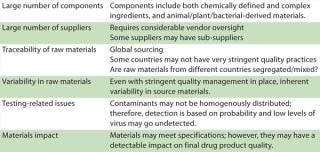
Table 1: Challenges related to raw materials ()
Appropriate sourcing and raw materials traceability is a key mandate in pharmaceutical production. That said, identifying the origin of some raw materials is much more complex than initially anticipated. Some raw materials and excipients used in pharmaceutical development and formulation are traded on the commodities market, which makes traceability challenging.
One example is gelatin: Raw materials that go into its manufacture (e.g., ruminant bones and hooves) are traded as commodities on the international market several times before they end up in a finished product (20). Potts reported that a vendor claimed that a selection antibiotic (intended for use in developing new master cell banks) had “no animal-derived raw materials.” After further investigation, it was determined that the media used to grow bacteria for producing the antibiotic contained several bovine derived constituents, including peptones, bile, skeletal muscle, bone marrow, and so forth (21). Rubino also described case histories that emphasize a need to focus on raw materials origin not only with ADMs, but also products derived from recombinant bacteria (22).
Another concern is the impact of increasing globalization on materials sourcing and (bio)pharmaceutical manufacturing. Some countries still do not have stringent quality practices and a well-developed regulatory framework.
Two approaches may be taken to minimize impacts associated with raw materials: prevention through assessment, inspection, and control of incoming raw materials; and intervention, in which raw materials may be processed to minimize viral safety threats. Some companies incorporate additional upstream barrier technologies such as γ–irradiation, UV-C inactivation and HTST (high-temperature-short-time) treatment of raw materials (23,24,25) Although incorporating of such upstream virus barriers is not mandated, proactive implementation of adequate virus barriers does provide some business insurance against virus contamination events. It is important to remember, however, that these methods must not, in any way, alter target protein yield, downstream process performance, or product quality.
Assessment of raw materials must extend beyond just material specifications and must include a supplier’s manufacturing processes, quality systems, and sourcing strategy. Supply chain management is a strategic enabler for pharmaceutical business performance. A pharmaceutical company is ultimately responsible for putting processes into place that ensure the control of outsourced activities and quality of purchased materials. Periodic audits provide only a snapshot. Table 2 describes critical steps in supply chain management.
Table 2: Critical steps in supply chain management
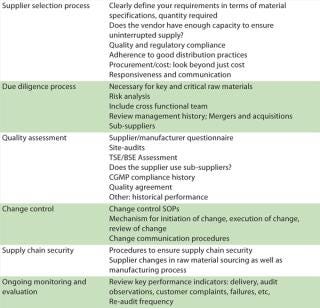
Table 2: Critical steps in supply chain management ()
Are Current Detection Methods Obsolete?
Short answer: No. Conventional viral infectivity assays were developed over a half-century ago, essentially for clinical virology applications. Although their specificity and sensitivity have not been systematically evaluated, they have stood the test of time (4). Newer methods offer more sophisticated tools and validate the utility of currently available tools. Alternative detection methods are acceptable if they have been shown to compare favorably with conventional methods in terms of specificity and sensitivity (26).
Whereas conventional methods for virus detection have stood the test of time, newer methods provide us with an upgraded lexicon. That allows us to query the complex world of genomics and protreomics by applying sophisticated computational and bioinformatics tools.
Newer Detection Methods: Are They Ready for Primetime?
Several advanced methodologies have become available, including massive parallel (deep) sequencing, degenerative polymerase chain reaction (PCR) mass spectrometry, and panmicrobial microarrays. The significant strengths of such methods lie in their exquisite sensitivity and specificity, as well as their ability to screen for a wide variety of agents in a single test. It is necessary to recognize, however, that detection of a genome (or parts thereof) does not correspond to presence of an infectious agent. Conventional infectivity assays rely on replication of the agent in a permissive system (be it tissue culture or in vivo tests) to detect a replication-competent virus. Molecular techniques detect the genome as a surrogate marker.
The high sensitivity of newer methods must be tempered with caution. Use newer methods to define the universe of contaminants and then scientifically assess their relevance. If the sequences match the viral database (VDB), it is necessary to evaluate the significance of the probable viral hit to determine whether there could be a nonviral source for that hit.
Currently, newer methods can supplement rather than replace conventional methods. They are not ready yet for use as first-line quality control. They need to be validated. Moreover, the newer methods require that appropriate reference materials be available (26). Their applications could potentially include characterization of cell banks for new cell substrates or for cases in which the history of the cell line and reagents used in establishment are unclear, as well as characterization of animal-derived raw material (27).
Novel Cell Substrates
Mammalian cell culture has become the accepted method of production for monoclonal antibodies and other recombinant proteins. The Chinese hamster ovary (CHO) system is the workhorse of the biotech industry. It is reportedly used for as much as 80% of products.
But novel cell substrates are also being introduced. Their use carries with it a concomitant risk. Concerns are related to unknown viruses, unexpecte
d viruses, and novel viruses (28). Although plant viruses and viroids are not known to replicate in humans and animals, they can replicate in insect vectors (cross kingdom) (29). Novel systems will require a better understanding of the cell substrate. New adventitious agents in novel systems will require new susceptible cell lines (30).
Although virus clearance evaluation (validation) approaches may be similar to those currently used, some questions to be addressed when using novel cell substrates include the virus validation panel to be used and the level of clearance/safety factor to be incorporated.
Viral Safety Assurance: A Moving Target
The diversity of the origins of biological materials, the introduction of novel production systems, and the high number of known and unknown viruses makes virus safety assurance a moving target. In addition, increased globalization — not just with international travel but also trade and commerce that affects raw materials and supply chain management — will continue to make us vulnerable to emerging and existing viruses.
Anecdotal evidence and systematic assessments have attested to the fact that biotech products have had an excellent safety record. This can largely be attributed to the viral safety tripod strictly adhered to in the industry: adequate sourcing, documentation of virus clearance evaluation (virus validation studies), and in-process testing. Newer anlaytical methods have demonstrated the robustness of conventional detection methods in ensuring virus safety. Nevertheless, testing gaps and alternative methods should be evaluated to continue to ensure safe, high-quality human biologicals (from a virological safety standpoint) (31).
Risk assessment, mitigation, and management are key components of the quality risk management paradigm. Newer test methods have yet to be validated; use them to supplement rather than replace conventional methods, to define the universe of contaminants, and then scientifically assess the relevance of data. Knowledge management is an enabler of the quality system. Recognize that: “The weakest link in the chain is also the strongest. It can break the chain.” A virus safety management program based on scientific reasoning, benefit-risk assessment, and knowledge management rather than heuristics is imperative for maintaining a high level of virus safety assurance.
About the Author
Author Details
Hazel Aranha, PhD, RAC is manager of viral clearance and safat Catalent Pharma Solutions, Morrisville, NC; [email protected].
REFERENCES
1.) PhRMA 2011.Biotechnology Medicines in Development, Pharmaceutical Research and Manufacturers of America, Washingtion.
2.) DiMasi, JA, L Feldman, and A Seckler. 2010. Trends in Risks Associated with New Drug Development: Success Rates for Investigational drugs. Clin. Pharmacol. Ther. 87:272-277.
3.) Ludwig, B.High-Development Costs, Complex Manufacturing, and Legal Hurdles Are Holding Back Generic Drugmakers Seeking to Copy Pricey Biotech Medications Nearing the End of Their Patents Reuters.
4.) Wiebe, ME 2011.. Conventional Virus Assays and the Need for New Technologies.
5.) 1999.ICH Q5A (R1): Viral Safety Evaluation of Biotechnology Products Derived From Cell Lines of Human or Animal Origin US Fed. Reg:9-10.
6.) Bethencourt, V. 2009. Virus Stalls Genzyme Plant. Nat. Biotech. 27:681.
7.) Kerr, A, and R. Nims. 2010. Adventitious Viruses Detected in Biopharmaceutical Bulk Harvest Samples Over a 10-Year Period. PDA J. Pharm. Sci. Technol. 64:481-485.
8.) Moody, M. 2010.. MMV Contamination, a Case Study: Detection, Root Cause Determination and Corrective Actions.
9.) Skrine, J. 2010.. A Biotech Production Facility Contamination Case Study — Mouse Minute Virus.
10.) Pastoret, PP. 2010. Human and Animal Vaccine Contaminations. Biologicals 38:332-334.
11.) Woolhouse, ME. 2008. Temporal Trends in the Discovery of Human Viruses. Proc. Biol. Sci. 275:2111-2115.
12.) Meehan, BM. 1997. Sequence of Porcine Circovirus DNA: Affinities with Plant Circoviruses. J. Gen. Virol. 78:221-227.
13.) Cool, M. 2011. No Detectable X MRV in Subjects with Chronic Fatigue Syndrome from Quebec. Virology 420:66-72.
14.) Simon, F, H Savini, and P. Parola. 2008. Chikungunya: A Paradigm of Emergence and Globalization of Vector-Borne Diseases. Med. Clin. North Am. 92:1323-1343.
15.) Boneva, RS, and TM. Folks. 2004. Xenotransplantation and Risks of Zoonotic. Infections. Ann. Med. 36:504-517.
16.) Yamanouchi, K. 2000. Potential Risk of Xenotransplant-Associated Infections. Transplant Proc. 32:1155-1156.
17.) Aranha, H. 2005. Virological Safety of Biopharmaceuticals. A Risk-Based Approach. Bioprocess Int. 4:S17-S20.
18.) Lower, J. 2011.. Risks Associated with Cell Substrates and Other Biological Materials and Product Safety.
19.) Tagmyer, T. 2011.. Evaluating Safety of Vendor Sourced Raw Materials Used in Vaccine Manufacturing.
20.) Mackay, D, and N. Kriz. 2010. Current Challenges in Viral Safety and Extraneous Agent Testing. Biologicals 38:335-337.
21.) Potts, BJ. 2010. TSE Case Studies Associated with Japanese and Other Regulatory Authoritie: Talk Transcript. PDA J. Pharm. Sci. Technol. 64:442-444.
22.) Rubino, MJ. 2010. Raw Materials Case Histories. PDA J. Pharm. Sci. Technol. 64:440-441.
23.) Dehghani, H. 2011.. State of the Art for Monitoring and Ensuring Biosafety in Manufacturing.
24.) Gauvin, G, and R Nims. 2010. Gamma Irradiation of Serum for the Inactivation of Adventitious Contaminants. PDA J. Pharm. Sci. Technol. 64:432-435.
25.) Weaver, B, and S. Rosenthal. 2010. Viral Risk Mitigation for Mammalian Cell Culture Media. PDA J. Pharm. Sci. Technol. 64:436-439.
26.) Motitschke, A, HP Ottiger, and C. Jungback. 2010. Evaluation of the Sensitivity of PCR Methods for the Detection of Extraneous Agents and Comparison with In Vivo Testing. Biologicals 38:389-392.
27.) Lubiniecki, AS. 2011.. Closing Remarks.
28.) Khan, AS. 2011.. Investigations of New Technologies for Latent Virus Detection.
29.) Hammond, R. 2011.. Risks Associated with Plant Viruses and Viroids.
30.) Stacey, G. 2011.. New WHO Guidance on Evaluation of Cell Substrates and Considerations for Insect Cell Substrates.
31.) Marcus-Sekura, C. 2011. Evaluation of the Human Host Range of Bovine and Porcine Viruses that May Contaminate Bovine Serum and Porcine Trypsin Used in the Manufacture of Biological Products. Biologicals 39:359-369.
You May Also Like